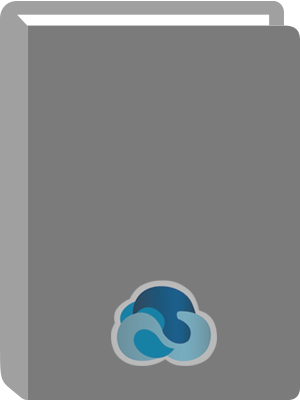
Synthetic Methods for Biologically Active Molecules : Exploring the Potential of Bioreductions.
Title:
Synthetic Methods for Biologically Active Molecules : Exploring the Potential of Bioreductions.
Author:
Brenna, Elisabetta.
ISBN:
9783527665815
Personal Author:
Edition:
1st ed.
Physical Description:
1 online resource (407 pages)
Contents:
Synthetic Methods for Biologically Active Molecules: Exploring the Potential of Bioreductions -- Contents -- Preface -- List of Contributors -- 1 Development of Sustainable Biocatalytic Reduction Processes for Organic Chemists -- 1.1 Introduction -- 1.2 Biocatalytic Reductions of C=O Double Bonds -- 1.2.1 Biocatalytic Reductions of Ketones to Alcohols -- 1.2.2 Biocatalytic Reductions of Aldehydes to Alcohols -- 1.2.3 Biocatalytic Reductions of Carboxylic Acids to Aldehydes -- 1.2.4 Biocatalytic Reductions of Carboxylic Acids to Alcohols -- 1.3 Biocatalytic Reductions of C=C Double Bonds -- 1.4 Biocatalytic Reductions of Imines to Amines -- 1.5 Biocatalytic Reductions of Nitriles to Amines -- 1.6 Biocatalytic Deoxygenation Reactions -- 1.7 Emerging Reductive Biocatalytic Reactions -- 1.8 Reaction Engineering for Biocatalytic Reduction Processes -- 1.9 Summary and Outlook -- References -- 2 Reductases: From Natural Diversity to Established Biocatalysis and to Emerging Enzymatic Activities -- 2.1 Reductases: Natural Occurrence and Context for Biocatalysis -- 2.2 Emerging Cases of Reductases in Biocatalysis -- 2.2.1 Motivation: The Quest for Novel Enzymes and Reactivities -- 2.2.2 Imine Reductases -- 2.2.3 Nitrile Reductases: The Next Member in the Portfolio of Reductases? -- 2.2.4 Other Emerging N-Based Enzymatic Reductions: Nitroalkenes and Oximes -- 2.2.5 From Carboxylic Acids to Alcohols: Biocatalysis -- 2.3 Concluding Remarks -- References -- 3 Synthetic Strategies Based on C=C Bioreductions for the Preparation of Biologically Active Molecules -- 3.1 Introduction -- 3.2 Bioreduction of α,β-Unsaturated Carbonyl Compounds -- 3.2.1 Aldehydes -- 3.2.2 Ketones -- 3.3 Bioreduction of Nitroolefins -- 3.4 Bioreduction of a,b-Unsaturated Carboxylic Acids and Derivatives -- 3.4.1 Monoesters and Lactones -- 3.4.2 Diesters -- 3.4.3 Carboxylic Acids.
3.4.4 Anhydrides and Imides -- 3.5 Bioreduction of a,b-Unsaturated Nitriles -- 3.6 Concluding Remarks -- References -- 4 Synthetic Strategies Based on C=O Bioreductions for the Preparation of Biologically Active Molecules -- 4.1 Introduction -- 4.2 Synthesis of Biologically Active Compounds through C=O Bioreduction -- 4.2.1 Keto Esters -- 4.2.1.1 α-Keto Esters -- 4.2.1.2 β-Keto Esters -- 4.2.1.3 Other Keto Esters -- 4.2.2 Diketones -- 4.2.3 α-Halo Ketones -- 4.2.4 (Hetero)Cyclic Ketones -- 4.2.5 "Bulky-Bulky" Ketones -- 4.2.6 Miscellaneous -- 4.3 Other Strategies to Construct Biologically Active Compounds -- 4.4 Summary and Outlook -- References -- 5 Protein Engineering: Development of Novel Enzymes for the Improved Reduction of C=C Double Bonds -- 5.1 Introduction -- 5.2 The Protein Engineering Process and Employed Mutagenesis Methods -- 5.3 Examples of Rational Design of Old Yellow Enzymes -- 5.4 Evolving Old Yellow Enzymes (OYEs) -- 5.4.1 Evolving OYE1 as a Catalyst in the Stereoselective Reduction of 3-Alkyl-2-cyclohexenone Derivatives and Baylis-Hillman Adducts -- 5.4.2 Evolving the Pentaerythritol Tetranitrate (PETN) Reductase as a Catalyst in the Reduction of α,β-Unsaturated Carbonyl Compounds and E-Nitroolefins -- 5.4.3 Evolving Nicotinamide-Dependent 2-Cyclohexenone Reductase (NCR) from Zymomonas mobilis for the Reduction of α,β-Unsaturated Ketones -- 5.4.4 Evolving the YqjM from Bacillus subtilis for Enhanced Activity, Substrate Scope, and Stereoselectivity in the Reduction of α,β-Unsaturated Ketones -- 5.5 Conclusions and Perspectives -- References -- 6 Protein Engineering: Development of Novel Enzymes for the Improved Reduction of C=O Double Bonds -- 6.1 Introduction -- 6.2 Detailed Characterization of PAR -- 6.2.1 Location of PAR in Styrene Metabolic Pathway -- 6.2.2 Physicochemical Properties of PAR -- 6.2.3 Enzymatic Properties of PAR.
6.2.4 Docking Model Construction of PAR -- 6.3 Detailed Characterization of LSADH -- 6.3.1 Screening of LSADH from Styrene-Assimilating Soil Microorganisms -- 6.3.2 Physicochemical Properties of LSADH -- 6.3.3 Enzymatic Properties of LSADH -- 6.4 Engineering of PAR for Increasing Activity in 2-Propanol/Water Medium -- 6.4.1 Construction of Sar268 Mutant -- 6.4.2 Construction of HAR1 Mutant -- 6.4.3 Characterization of Sar268 and HAR1 -- 6.5 Application of Whole-Cell Biocatalysts Possessing Mutant PARs and LSADH -- 6.5.1 E. coli Whole-Cell Biocatalysts Possessing Mutant PARs and LSADH -- 6.5.2 Application of Immobilized E. coli Whole-Cell Catalysts to Continuous Production of Chiral Alcohol -- 6.5.3 Application of Immobilized E. coli Whole-Cell Catalysts (LASDH) for Regenerating NADH with IPA -- 6.6 Engineering of b-Keto Ester Reductase (KER) for Raising Thermal Stability and Stereoselectivity -- 6.6.1 Enzymatic Properties of KER -- 6.6.2 Engineering of KER and Characterization of Mutant Enzymes -- 6.7 New Approach for Engineering or Obtaining Useful ADHs/ Reductases -- 6.7.1 Engineering the Coenzyme Dependency of Ketol-Acid Reductoisomerase (KARI) -- 6.7.2 Engineering Substrate- and Stereospecificity of Reductases by Structure-Guided Method -- 6.7.3 Engineering Database: Systematic Information of Sequence-Structure-Function -- 6.7.4 Metagenomics -- References -- 7 Synthetic Applications of Aminotransferases for the Preparation of Biologically Active Molecules -- 7.1 Introduction -- 7.1.1 Aminotransferases -- 7.1.2 Transamination Reaction -- 7.1.3 Stereoselectivity of Aminotransferases -- 7.2 Applications -- 7.2.1 Biotransformation Process -- 7.2.2 Biologically Active Molecules -- 7.2.3 Process Economy -- 7.3 Challenges -- 7.3.1 Substrate Specificity -- 7.3.2 Improving Reaction Yield -- 7.3.3 Process Scale-Up -- 7.4 Future Research Needs.
7.5 Conclusions -- References -- 8 Strategies for Cofactor Regeneration in Biocatalyzed Reductions -- 8.1 Introduction: NAD(P)H as the Universal Reductant in Reduction Biocatalysis -- 8.2 The Most Relevant Cofactor Regeneration Approaches - and How to Choose the Most Suitable One -- 8.2.1 Electrochemical Regeneration of NAD(P)H -- 8.2.2 H2 as Reducing Agent -- 8.2.3 Formates as Reducing Agents -- 8.2.4 Phosphites as Stoichiometric Reductants -- 8.2.5 Alcohols as Stoichiometric Reductants -- 8.2.6 Glucose as Stoichiometric Reductant -- 8.3 Coupling the Reduction Reaction to a Regeneration Reaction Producing a Valuable Compound -- 8.4 Avoiding NAD(P)H: Does It Also Mean Avoiding the Challenge? -- 8.5 Conclusions -- References -- 9 Solvent Effects in Bioreductions -- 9.1 Introduction -- 9.2 Solvent Systems for Biocatalytic Reductions -- 9.2.1 Bioreduction in Aqueous Systems -- 9.2.2 Bioreduction in Monophasic Aqueous-Organic Systems -- 9.2.3 Bioreduction in Biphasic Aqueous-Organic Systems -- 9.2.4 Bioreduction in Micro- or Nonaqueous Systems -- 9.2.5 Bioreduction in Nonconventional Media -- 9.2.5.1 Ionic Liquids -- 9.2.5.2 Supercritical Fluids -- 9.2.5.3 Combining ILs and SFs -- 9.2.5.4 Gas-Phase Media -- 9.2.5.5 Reverse Micelles -- 9.3 Solvent Control of Enzyme Selectivity -- 9.4 Concluding Remarks -- References -- 10 Application of In situ Product Removal (ISPR) Technologies for Implementation and Scale-Up of Biocatalytic Reductions -- 10.1 Introduction -- 10.2 Process Requirements for Scale-Up -- 10.3 Bioreduction Process Engineering -- 10.4 In situ Product Removal -- 10.5 Biocatalyst Format -- 10.5.1 Whole-Cell Processes -- 10.5.2 Isolated Enzyme Processes -- 10.6 Selected Examples -- 10.6.1 ISPR with Resins -- 10.6.2 ISPR with Solvent Extraction -- 10.6.3 ISPR with Crystallization -- 10.6.4 Removal of Acetone -- 10.7 Future Outlook.
10.7.1 Protein Engineering -- 10.7.2 Choice of Methods -- 10.7.3 Process Integration -- 10.8 Conclusions -- References -- 11 Bioreductions in Multienzymatic One-Pot and Cascade Processes -- 11.1 Introduction -- 11.2 Coupled Oxidation and Reduction Reactions -- 11.3 Consecutive and Cascade One-Pot Reductions -- 11.4 Cascade Processes, Including Biocatalyzed Reductive Amination Steps -- 11.5 Other Examples of Multienzymatic Cascade Processes, Including Bioreductive Reactions -- References -- 12 Dynamic Kinetic Resolutions Based on Reduction Processes -- 12.1 Introduction -- 12.2 Cyclic Compounds -- 12.3 Acyclic α-Substituted-β-Keto Esters and 2-Substituted-1,3- Diketones -- 12.4 Acyclic Ketones and Aldehydes -- 12.5 Conclusions -- References -- 13 Relevant Practical Applications of Bioreduction Processes in the Synthesis of Active Pharmaceutical Ingredients -- 13.1 Introduction -- 13.2 Ketoreductases -- 13.2.1 Ethyl 4-chloro-3-hydroxybutanoate -- 13.2.2 Atorvastatin -- 13.2.3 Montelukast -- 13.2.4 Ramatroban -- 13.2.5 Ezetimibe -- 13.2.6 Profens -- 13.2.7 Atazanavir -- 13.2.8 Chemokine Receptor Inhibitor -- 13.2.9 Duloxetin -- 13.2.10 6-Hydroxybuspirone -- 13.2.11 LY 300164 -- 13.2.12 Paclitaxel -- 13.3 Ene Reductases -- 13.3.1 Levodione -- 13.3.2 (+)-Dihydrocarvone -- 13.3.3 Butyrolactone - Jasplakinolide and Amphidinolides -- 13.3.4 (R )-Flurbiprofen -- 13.3.5 Ethyl (S )-2-ethoxy-3-(4-methoxyphenyl)propanoate - Tesaglitazar -- 13.3.6 Methyl (Z)-2-bromocrotonate - Antidiabetic Drug Candidates -- 13.3.7 Roche Ester -- 13.3.8 Human Neurokinin-1 Receptor Antagonists -- 13.3.9 Asymmetric Synthesis of Amino Acid Derivatives -- 13.4 Others -- 13.4.1 Amino Acid Dehydrogenase-Catalyzed Processes -- 13.4.1.1 Saxagliptin -- 13.4.1.2 Omapatrilat -- 13.4.1.3 Inogatran -- 13.4.1.4 Corticotropin-releasing Factor-1 (CRF-1) Receptor Antagonist -- 13.4.1.5 AG7088.
13.4.2 Pyrrolo[2,1-c][1,4]benzodiazepines (Antitumor Agents).
Abstract:
This useful reference focuses on the currently available toolbox of bio-catalysed reductions of C=O, C=C and formal C=N bonds to show which transformations can be reliably used in manufacturing processes and which still require improvements. Following an introductory chapter, chapters 2-4 present the synthetic strategies that are currently available for the reduction of C=C and C=O bonds and for reductive amination, by means of whole-cell catalysts and isolated enzymes. Chapters 5-7 go on to describe the improvements achieved thus far, illustrating the current versatility of enzymes in organic synthesis. Chapters 8-12 present the improvements brought about by the optimization of reaction conditions, and the use of particular synthetic sequences. The final chapter describes practical applications of bio-reductions for the synthesis of active pharmaceutical ingredients. With its excellent and comprehensive overview, this book will be of great interest to those working in academia and industry. From the contents: * Development of Sustainable Biocatalyzed Reduction Processes for Organic Chemists * Reductases: From Natural Diversity to Biocatalysis and Emerging Enzymatic Activities. * Synthetic Strategies Based on C=C Bioreductions * Synthetic Strategies Based on C=O Bioreductions * Development of Novel Enzymes for the Improved Reduction of C=C Double Bonds * Development of Novel Enzymes for the Improved Reduction of C=O Double Bonds * Synthetic Applications of Aminotransferases * Strategies for Cofactor Regeneration in Biocatalyzed Reductions * Effects of Solvent System and Substrate Loading in Bioreduction * Perspectives in the Use of In-Situ Product Removal (ISPR) Techniques in Bioreductions * Multi-Enzymatic Cascade Reactions Based on Reduction Processes * Relevant Practical Applications of Bioreduction Processes in the Synthesis of Active
Pharmaceutical Ingredients.
Local Note:
Electronic reproduction. Ann Arbor, Michigan : ProQuest Ebook Central, 2017. Available via World Wide Web. Access may be limited to ProQuest Ebook Central affiliated libraries.
Genre:
Electronic Access:
Click to View