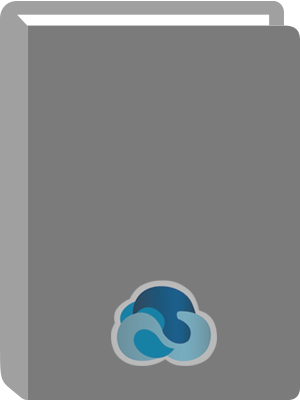
Rechargeable Sensor Networks : Technology, Theory, and Application - Introducing Energy Harvesting to Sensor Networks.
Title:
Rechargeable Sensor Networks : Technology, Theory, and Application - Introducing Energy Harvesting to Sensor Networks.
Author:
Chen, Jiming.
ISBN:
9789814525466
Personal Author:
Physical Description:
1 online resource (370 pages)
Contents:
Contents -- Preface -- 1. Wind Energy Harvesting for Recharging Wireless Sensor Nodes: Brief Review and A Case Study -- 1. Introduction -- 2. Wind Energy Harvesting from Wind Turbines -- 2.1. Description of technique -- 2.1.1. Savonius wind turbine -- 2.1.2. Darrieus wind turbine -- 2.1.3. Maximum available power -- 2.1.4. Efficiency -- 2.2. Examples -- 3. Energy Harvesting from Flow-Induced Vibration -- 3.1. Description of technique -- 3.2. Examples -- 3.2.1. Karman vortex street -- 3.2.2. Flapping -- 4. Energy Harvesting from Helmholtz Resonators -- 4.1. Description of technique -- 4.2. Examples -- 5. Comparisons -- 6. A Case Study on a Novel Method of Harvesting Wind Energy Through Piezoelectric Vibration for Low-Power Autonomous Sensors -- 6.1. Piezoelectric wind harvester -- 6.1.1. Characteristic of wind flow -- 6.1.2. Characteristic of piezoelectric effect -- 6.1.3. Characteristic of piezoelectric wind harvester -- 6.1.4. Performance of the piezoelectric wind harvester -- 6.2. Power storage, supply, and regulating circuit -- 6.3. Experimental Results -- 7. Conclusions -- Acknowledgments -- References -- 2. Rechargeable Sensor Networks with Magnetic Resonant Coupling -- 1. Introduction -- 1.1. Magnetic resonant coupling: A primer -- 1.2. Chapter organization -- 2. Single-Node Charging for a Sparse WSN -- 2.1. Problem description -- 2.2. Renewable energy cycles -- 2.3. Optimal traveling path -- 2.4. Problem formulation -- 2.5. An initial transient cycle -- 2.6. An example -- 3. Multinode Charging for a Dense WSN -- 3.1. Mathematical modeling -- 3.2. Problem formulation and properties -- 3.3. An example -- 4. Bundling Mobile Base Station and Magnetic Resonant Coupling -- 4.1. Mathematical modeling and problem formulation -- 4.2. Downsizing solution space: A location-based formulation -- 4.3. An example -- 5. Summary -- Acknowledgments.
References -- 3. Cross-Layer Resource Allocation in Energy-Harvesting Sensor Networks -- 1. Introduction -- 2. Static Resource Allocation with Renewable Energy -- 2.1. Rate, bandwidth, and flow allocation with renewable energy -- 2.2. Energy-aware routing with renewable energy -- 3. Dynamic Resource Allocation with Renewable Energy -- 3.1. Basic performance limits and tradeoffs with renewable energy -- 3.2. Finite horizon power allocation with renewable energy -- 3.3. A cross-layer framework for multihop networks with renewable energy -- 3.3.1. Multihop rate control (MRC) -- 3.3.2. Multihop power allocation (MPA) -- 3.3.3. Multihop routing -- 3.4. Summary -- 4. Conclusions -- Acknowledgments -- References -- 4. Energy-Harvesting Technique and Management for Wireless Sensor Networks -- 1. Introduction -- 2. Energy-Harvesting Module -- 3. Design of Solar-Harvesting Module -- 3.1. Design challenges -- 3.1.1. Solar energy -- 3.1.2. Energy storage characteristics -- 3.1.3. Sensor load -- 3.2. Solar Mote module -- 3.2.1. General mechanism of energy-harvesting modules -- 3.2.2. Overview of Solar Mote module -- 3.2.3. Energy flow control -- 3.3. Hardware design and operation principle of Solar Mote module -- 3.3.1. System description -- 3.3.2. Hardware design -- 3.3.3. Control switch -- 3.3.4. Solar Mote controller -- 3.3.5. External sensor node -- 3.4. Experimental evaluation of Solar Mote module -- 3.4.1. Software design -- 3.4.2. Charging and discharging measurement -- 3.4.3. Short-term experiment -- 3.4.4. Long-term experiment -- 4. Energy Management in Energy-Harvesting WSNs -- 4.1. MPPT and energy neutral operation -- 4.1.1. MPP tracking -- 4.1.2. Adaptive duty cycling for energy-harvesting sensor networks (energy neutral operation) -- 4.1.3. Harvested energy prediction -- 5. Duty Cycling under Energy Constraint.
5.1. Duty cycling under energy constraint -- 5.2. Dynamic node activation for target monitoring -- 5.2.1. Network model -- 5.2.2. Recharging and discharging model -- 5.2.3. Utility function -- 5.2.4. The hardness of the problem -- 5.3. Node active policy for target monitoring -- 5.3.1. When ρ > 1 -- 5.3.1.1. Linear programming solution -- 5.3.1.2. Greedy hill-climbing activation scheme -- 5.3.2. When ρ ≤ 1 -- 5.4. Experimental results and evaluation -- 5.4.1. Charging pattern measurement -- 5.4.2. Evaluation of the greedy hill-climbing algorithms -- 6. Summary -- Acknowledgments -- References -- 5. Information Capacity of an AWGN Channel Powered by an Energy-Harvesting Source -- 1. Introduction -- 2. Related Work -- 3. Capacity of an AWGN Channel with an Energy-Harvesting Transmitter -- 4. Capacity with Processor Energy (PE) -- 5. Achievable Rate with Energy Inefficiencies -- 6. Fading AWGN Channel -- 6.1. Capacity with energy consumption in sensing and processing -- 6.2. Achievable rate with energy inefficiencies -- 7. Combining Information and Queuing Theory -- 8. Finite Buffer -- 9. Multiple Access Channel -- 9.1. Finite buffer -- 9.2. GMAC with finite buffer, infinite buffer combination -- 10. Conclusions -- Appendix A. Proof of Theorem1 -- References -- 6. Energy Harvesting in Wireless Sensor Networks -- 1. Introduction -- 2. Overview of a Sensor Node -- 2.1. Power unit -- 2.2. Sensing unit -- 2.3. Processing unit -- 2.4. Transceiver unit -- 3. Energy Harvesting -- 3.1. Concept -- 4. Benefits and Drawbacks -- 5. Energy-Harvesting Management -- 5.1. Duty cycle for perpetual operation -- 5.2. Adaptive sensing -- 6. Discussion -- Bibliography -- 7. Topology Control for Wireless Sensor Networks and Ad Hoc Networks -- 1. Overview -- 1.1. Topology -- 1.2. Network -- 2. Network Topology -- 2.1. Wired networks -- 2.2. Wireless networks.
3. Need for Topology Control -- 4. Graph Theory-Based Approach -- 4.1. Graphs in network -- 4.2. Graph theory-based solutions -- 5. Algorithms, Dominating Set and Minimum Connected Dominating Set, Optimization Algorithms -- 5.1. Maximum independent set (MIS)-based methods -- 5.2. Multi-point relay (MPR)-based methods -- 6. Cross-Layer-Based Approach -- 7. Future Research Direction -- References -- 8. An Evolutionary Game Approach for Rechargeable Sensor Networks -- 1. Introduction -- 2. Model -- 2.1. Success probability -- 2.2. Transition probabilities -- 2.3. Initial energy level -- 3. Properties of the Fitness -- 4. Evolutionary Stable Strategies -- 4.1. Nash equilibrium -- 4.2. Definition of a standard evolutionary game -- 4.3. ESS in the semi-dynamic game -- 5. Computing the Equilibrium -- 5.1. With breakdown -- 5.2. Without breakdown -- 6. What About Recharging? -- 6.1. With breakdown -- 6.2. Without breakdown -- 7. Dynamics -- 7.1. Replicator dynamics -- 7.2. Brown-von Neumann-Nash dynamics -- 8. Numerical Results -- 8.1. The Hawk and Dove resource abundance paradox -- 8.2. The initial energy paradox -- 8.3. Dynamics -- 9. Discussion and Conclusions -- References -- 9. Marine Sediment Energy Harvesting for Sustainable Underwater Sensor Networks -- 1. Introduction -- 2. Marine Sediment Energy Harvesting via MFCs -- 2.1. Principle of biomass-based energy harvesting -- 2.2. Computational model of MFCs -- 3. Design of Marine Sediment MFCs -- 3.1. Single-chamber marine MFC (SCM-MFC) -- 3.2. Distributed marine MFC (DMFC) -- 4. Power Management and System Integration with MFCs -- 5. Conclusions -- References -- 10. Wireless Rechargeable Sensor Networks in the Smart Grid -- 1. Introduction -- 2. Smart Grid Monitoring with Wireless Rechargeable Sensor Networks -- 3. RF Energy-Harvesting Basics.
4. RF Energy Harvesting for Wireless Rechargeable Sensor Networks for Smart Grid Deployments -- 4.1. Sustainable wireless rechargeable sensor network (SuReSense) -- 4.1.1. Landmark selection -- 4.1.2. Clustering and path formation -- 4.2. Differentiated RF power transmission (DRIFT) -- 4.3. Mission-aware placement of wireless power transmitters (MAPIT) -- 5. Performance Evaluation -- 5.1. Performance comparison of SuReSense and DRIFT -- 5.2. Performance evaluation of MAPIT -- 6. Summary and Open Issues -- References -- 11. Energy-Harvesting Methods for Medical Devices -- 1. Introduction -- 1.1. Sensor networks -- 2. Harvesting Methods -- 2.1. Mechanical energy -- 2.1.1. Electromagnetic method -- 2.1.2. Piezoelectric method -- 2.1.3. Electrostatic method -- 2.2. Thermal energy -- 2.3. Energy harvesting outside the body -- 2.3.1. Potential human power sources -- 2.4. Energy harvesting inside the body -- 2.4.1. Potential human power sources -- 2.4.2. Technology Readiness Level assessment -- 3. Medical Applications -- 3.1. Scenarios for medical application -- 3.2. Biomechanical harvesting energy -- 3.3. Cardiac implant applications -- 3.4. Smartphones -- 3.5. Thermoelectric generator in wearable devices -- 3.6. Thermoelectric generator in clothing -- 4. Current Status and Future Trends -- 4.1. Energy sources -- 4.1.1. Microbatteries -- 4.1.2. Micro fuel cells -- 4.2. Ultra-low-power (ULP) systems -- 4.3. Power management (PM) -- 4.4. Technology Roadmap -- 4.4.1. Energy harvesting outside the body -- 4.4.2. Energy harvesting inside the body -- 5. Conclusions -- References -- Index.
Abstract:
The harvesting of energy from ambient energy sources to power electronic devices has been recognized as a promising solution to the issue of powering the ever-growing number of mobile devices around us. Key technologies in the rapidly growing field of energy harvesting focus on developing solutions to capture ambient energy surrounding the mobile devices and convert it into usable electrical energy for the purpose of recharging said devices. Achieving a sustainable network lifetime via battery-aware designs brings forth a new frontier for energy optimization techniques. These techniques had, in their early stages, resulted in the development of low-power hardware designs. Today, they have evolved into power-aware designs and even battery-aware designs. This book covers recent results in the field of rechargeable sensor networks, including technologies and protocol designs to enable harvesting energy from alternative energy sources such as vibrations, temperature variations, wind, solar, and biochemical energy and passive human power.
Local Note:
Electronic reproduction. Ann Arbor, Michigan : ProQuest Ebook Central, 2017. Available via World Wide Web. Access may be limited to ProQuest Ebook Central affiliated libraries.
Genre:
Electronic Access:
Click to View