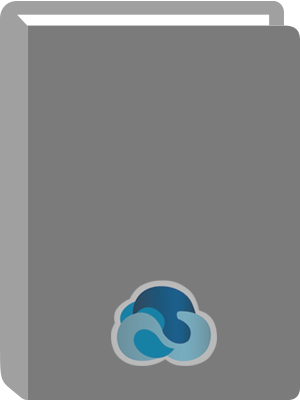
Nanocarbons for Advanced Energy Conversion.
Title:
Nanocarbons for Advanced Energy Conversion.
Author:
Feng, Xinliang.
ISBN:
9783527680047
Personal Author:
Edition:
1st ed.
Physical Description:
1 online resource (329 pages)
Contents:
Cover -- Title Page -- Copyright -- Contents -- List of Contributors -- Preface -- Chapter 1 Heteroatom-Doped Carbon Nanotubes as Advanced Electrocatalysts for Oxygen Reduction Reaction -- 1.1 Introduction -- 1.2 Experimental Evaluation of Electrocatalytic Activity toward ORR -- 1.3 Doped Carbon Nanotubes for ORR -- 1.3.1 Carbon Nanotubes Doped with Nitrogen -- 1.3.2 Carbon Nanotubes Doped with Heteroatoms Other Than Nitrogen -- 1.4 Conclusions -- Acknowledgments -- References -- Chapter 2 Doped Graphene as Electrocatalysts for Oxygen Reduction Reaction -- 2.1 Introduction -- 2.2 Active Sites and Mechanisms of ORR on Doped Graphene -- 2.2.1 ORR Mechanism on Doped Graphene -- 2.2.2 The Active Site of Doped Graphene for ORR -- 2.3 Synthesis and Performance of Doped Graphene -- 2.3.1 Nitrogen-Doped Graphene -- 2.3.2 Synthesis and Performance of Other Heteroatom-Doped Graphene -- 2.3.2.1 B-Doped Graphene -- 2.3.2.2 S-Doped Graphene -- 2.3.2.3 P and Other Heteroatom-Doped Graphene -- 2.4 Conclusions and Perspective -- References -- Chapter 3 Heteroatom-Doped Nanoporous Carbon for Electrocatalysis -- 3.1 Introduction -- 3.2 Synthesis of Doped Nanoporous Carbons -- 3.2.1 Synthesis of Heteroatom-Doped Ordered Mesoporous Carbons -- 3.2.1.1 Self-Assembling of Heteroatom-Rich Carbon Precursors through a Soft-Templating Method -- 3.2.1.2 Posttreatment of Ordered Mesoporous Carbon Framework with Heteroatom-Rich Chemicals -- 3.2.1.3 Hard-Templating Method with One-Step Doping Using Heteroatom-Rich Carbon Precursors -- 3.2.2 Synthesis of Doped Porous Graphene -- 3.2.2.1 Vapor-Assisted Method -- 3.2.2.2 Liquid-Phase Method -- 3.3 Heteroatom-Doped Nanoporous Carbons for Electrocatalysis -- 3.3.1 Oxygen Reduction Reaction (ORR) -- 3.3.2 Doped Ordered Mesoporous Carbon for ORR -- 3.3.3 Doped Graphene for ORR -- 3.3.3.1 Single Heteroatom-Doped Graphene.
3.3.3.2 Dual-Doped Graphene -- 3.3.3.3 Doped Graphene-Based Nanocomposites -- 3.3.4 Other Electrochemical Systems -- 3.4 Summary and Perspectives -- References -- Chapter 4 Nanocarbon-Based Nonprecious-Metal Electrocatalysts for Oxygen Reduction in Various Electrolytes -- 4.1 Introduction -- 4.2 Oxygen Reduction in Acidic Media -- 4.2.1 Heat-Treated Macrocyclic Compounds -- 4.2.2 Heat-Treated Nonmacrocyclic Catalysts -- 4.2.2.1 Nitrogen Precursors -- 4.2.2.2 Type of Transition Metals -- 4.2.2.3 Effect of Supports -- 4.2.2.4 Heating Temperatures -- 4.2.3 Importance of in situ Formed Graphitic Nanocarbons -- 4.3 Oxygen Reduction in Alkaline Media -- 4.3.1 Metal-Free Carbon Catalysts -- 4.3.1.1 Nitrogen-Doped Carbon -- 4.3.1.2 Boron and Sulfur Doping -- 4.3.1.3 Binary and Ternary Dopants -- 4.3.2 Heat-Treated M-N-C (M: Fe, Co) Catalysts -- 4.3.3 Nanocarbon/Transition Metal Compound Hybrids -- 4.4 Oxygen Reduction in Nonaqueous Li-O_2 Batteries -- 4.5 Summary and Perspective -- Acknowledgments -- References -- Chapter 5 Spectroscopic Analysis of Nanocarbon-Based non-precious Metal Catalyst for ORR -- 5.1 Introduction -- 5.2 Raman Spectroscopy -- 5.2.1 Theory -- 5.2.2 Characterization of Me-N-C Catalysts by Raman Spectroscopy -- 5.3 X-Band Electron Paramagnetic Resonance (EPR) Spectroscopy -- 5.3.1 Theory -- 5.3.2 Examples of EPR Spectroscopy in the Characterization of Me-N-C -- 5.4 X-ray-Induced Photoelectron Spectroscopy (XPS) -- 5.4.1 Theory -- 5.4.2 Example of Postmortem Analysis of Me-N-C Catalysts by XPS -- 5.5 Mossbauer Spectroscopy (MBS) -- 5.5.1 Theory -- 5.5.2 Effect of Iron Carbide Formation on the Concentration of Active Sites -- 5.5.3 Influence of the Electronic Structure: Correlation of Isomer Shift and TOF -- 5.6 X-ray Absorption Spectroscopy (XANES/EXAFS) of Metal Edges -- 5.6.1 Theory.
5.6.2 Influence of Preparation Parameters on the Next Neighbors (EXAFS) -- 5.6.3 Influence of the Pyrolysis Temperature on the Structure (XANES) -- 5.6.4 Correlation between XANES and Mossbauer Results -- 5.7 Possibilities to Do in situ Measurements (Coupled with an Electrochemical Cell/FC) -- 5.7.1 In situ XANES Spectroelectrochemistry on PANI-Fe-C Catalysts -- 5.8 Outlook -- References -- Chapter 6 Graphene as a Support for ORR Electrocatalysts -- 6.1 Introduction -- 6.2 Synthesis and Structural Characteristics of GNS-Supported Catalyst Nanoparticles (Me/GNS, Me = Mono or Bimetallic Catalysts) -- 6.3 Electrochemical Properties of Me Catalysts Supported on GNS-, Modified GNS-, and Hybrid GNS-Containing Materials -- 6.3.1 Electrocatalytic Activity of Me/GNS for Oxygen Reduction -- 6.3.2 Me Supported on Modified GNS -- 6.3.2.1 Functionalized Graphene by Thermal Exfoliation -- 6.3.2.2 Sulfonated Graphene -- 6.3.2.3 Nitrogen-Doped Graphene -- 6.3.2.4 Noncovalent Functionalized Graphene: PDDA-GNS, CTAB-GNS, and gds-DNA/rGO -- 6.3.3 Me Supported on Hybrid GNS-CB, GNS-CNT, and GNS-MeO_2 Materials -- 6.3.3.1 Me Supported on Hybrid GNS-CB -- 6.3.3.2 Me Supported on Hybrid GNS-CNT -- 6.3.3.3 Me Supported on GNS-MeO_2 Materials -- 6.4 Synthesis and Electrochemical Properties of Nanostructured Me Catalysts Supported on GNS -- 6.5 Conclusions -- References -- Chapter 7 Nanocarbons and Their Hybrids as Electrocatalysts for Metal-Air Batteries -- 7.1 Introduction -- 7.2 Nanocarbons -- 7.2.1 1D Carbon Nanomaterial -- 7.2.2 2D Carbon Nanomaterial -- 7.3 Nanocarbonaceous Electrocatalysts for Metal-Air Batteries -- 7.3.1 Metal-Free Nanocarbon Catalysts -- 7.3.2 Noble Metal-Nanocarbon Catalysts -- 7.3.3 Metal Oxide-Nanocarbon Catalysts -- 7.3.3.1 Mono-Metal Oxides -- 7.3.3.2 Mixed Metal Oxides -- 7.4 Conclusions and Future Perspectives -- Acknowledgments.
References -- Chapter 8 Nanocarbon-Based Hybrids as Cathode Electrocatalysts for Microbial Fuel Cells -- 8.1 Introduction to MFCs and MFC Cathodes -- 8.2 Nanocarbon-Supported Platinum Cathode Catalysts -- 8.3 Nanocarbon-Supported Precious-Metal-Free Cathode Catalysts -- 8.3.1 Transition Metal Macrocycles -- 8.3.2 Metal Oxide -- 8.3.3 Metal-Free Nanocarbon-Based Catalysts -- 8.3.4 Transition Metal-Containing Nanocarbon-Based Catalysts -- 8.4 Conclusions and Outlook -- References -- Chapter 9 Carbon Nanotubes and Graphene for Silicon-Based Solar Cells -- 9.1 Introduction -- 9.2 Carbon/Semiconductor Schottky Junction -- 9.3 Nanocarbon/Silicon Heterojunction Solar Cells -- 9.3.1 Theoretical Model -- 9.3.2 Chemical Doping -- 9.3.3 Antireflection Optimization -- 9.3.4 Hybrid Heterojunction and Photoelectrochemistry Solar Cells -- 9.3.5 Photodetectors -- 9.4 Summary -- References -- Chapter 10 Graphene as Transparent Electrodes for Solar Cells -- 10.1 Introduction -- 10.2 Production of Graphene -- 10.2.1 Micromechanical Cleavage -- 10.2.2 Liquid-Phase Exfoliation -- 10.2.3 Chemical Vapor Deposition -- 10.2.4 Graphene from Graphite Oxide -- 10.3 Optoelectronic Properties of Graphene -- 10.4 Transparent Conductive Films from Graphene -- 10.5 Organic Solar Cells -- 10.6 Hybrid Solar Cells -- 10.7 Dye-Sensitized Solar Cells -- 10.8 Summary and Future Perspectives -- References -- Chapter 11 Nanostructured Carbon Nitrides for Photocatalytic Water Splitting -- 11.1 Introduction -- 11.2 Photocatalytic Water Splitting -- 11.3 Graphitic Carbon Nitride for Photocatalytic Water Splitting -- 11.4 Nanostructure Design of Graphitic Carbon Nitride -- 11.4.1 Template-Assisted Method -- 11.4.1.1 Hard-Template Method -- 11.4.1.2 Soft-Template Method -- 11.4.2 Sulfur-Mediated Synthesis -- 11.4.3 Solvothermal Technology -- 11.4.4 Top-Down Strategy -- 11.4.5 Combined Methods.
11.5 Conclusions and Perspectives -- Acknowledgments -- References -- Index -- EULA.
Local Note:
Electronic reproduction. Ann Arbor, Michigan : ProQuest Ebook Central, 2017. Available via World Wide Web. Access may be limited to ProQuest Ebook Central affiliated libraries.
Genre:
Electronic Access:
Click to View