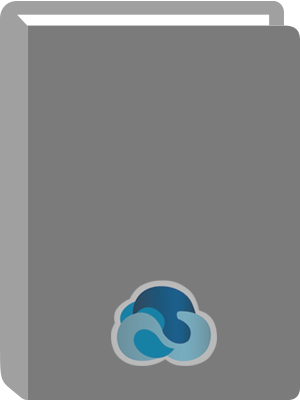
Supercapacitors : Materials, Systems and Applications.
Title:
Supercapacitors : Materials, Systems and Applications.
Author:
Lu, Max.
ISBN:
9783527646685
Personal Author:
Edition:
1st ed.
Physical Description:
1 online resource (569 pages)
Series:
New Materials for Sustainable Energy and Development Ser.
Contents:
Supercapacitors -- Contents -- Series Editor Preface -- Preface -- About the Series Editor -- About the Volume Editors -- List of Contributors -- 1 General Principles of Electrochemistry -- 1.1 Equilibrium Electrochemistry -- 1.1.1 Spontaneous Chemical Reactions -- 1.1.2 The Gibbs Energy Minimum -- 1.1.3 Bridging the Gap between Chemical Equilibrium and Electrochemical Potential -- 1.1.4 The Relation between E and DGr -- 1.1.5 The Nernst Equation -- 1.1.6 Cells at Equilibrium -- 1.1.7 Standard Potentials -- 1.1.8 Using the Nernst Equation - Eh-pH Diagrams -- 1.2 Ionics -- 1.2.1 Ions in Solution -- 1.2.1.1 Ion-Solvent Interactions -- 1.2.1.2 Thermodynamics -- 1.2.2 The Born or Simple Continuum Model -- 1.2.2.1 Testing the Born Equation -- 1.2.3 The Structure of Water -- 1.2.3.1 Water Structure near an Ion -- 1.2.3.2 The Ion-Dipole Model -- 1.2.3.3 Cavity Formation -- 1.2.3.4 Breaking up the Cluster -- 1.2.3.5 Ion-Dipole Interaction -- 1.2.3.6 The Born Energy -- 1.2.3.7 Orienting the Solvated Ion in the Cavity -- 1.2.3.8 The Leftover Water Molecules -- 1.2.3.9 Comparison with Experiment -- 1.2.3.10 The Ion-Quadrupole Model -- 1.2.3.11 The Induced Dipole Interaction -- 1.2.3.12 The Results -- 1.2.3.13 Enthalpy of Hydration of the Proton -- 1.2.4 The Solvation Number -- 1.2.4.1 Coordination Number -- 1.2.4.2 The Primary Solvation Number -- 1.2.5 Activity and Activity Coefficients -- 1.2.5.1 Fugacity (f') -- 1.2.5.2 Dilute Solutions of Nonelectrolytes -- 1.2.5.3 Activity (a) -- 1.2.5.4 Standard States -- 1.2.5.5 Infinite Dilution -- 1.2.5.6 Measurement of Solvent Activity -- 1.2.5.7 Measurement of Solute Activity -- 1.2.5.8 Electrolyte Activity -- 1.2.5.9 Mean Ion Quantities -- 1.2.5.10 Relation between f, y, andy -- 1.2.6 Ion-Ion Interactions -- 1.2.6.1 Introduction -- 1.2.6.2 Debye-Huckel Model for Calculating o2.
1.2.6.3 Poisson-Boltzmann Equation -- 1.2.6.4 Charge Density -- 1.2.6.5 Solving the Poisson-Boltzmann Equation -- 1.2.6.6 Calculation of D ui-I -- 1.2.6.7 Debye Length, K-1 or LD -- 1.2.6.8 The Activity Coefficient -- 1.2.6.9 Comparison with Experiment -- 1.2.6.10 Approximations of the Debye-Huckel Limiting Law -- 1.2.6.11 The Distance of Closest Approach -- 1.2.6.12 Physical Interpretation of the Activity Coefficient -- 1.2.7 Concentrated Electrolyte Solutions -- 1.2.7.1 The Stokes-Robinson Treatment -- 1.2.7.2 The Ion-Hydration Correction -- 1.2.7.3 The Concentration Correction -- 1.2.7.4 The Stokes-Robinson Equation -- 1.2.7.5 Evaluation of the Stokes-Robinson Equation -- 1.2.8 Ion Pair Formation -- 1.2.8.1 Ion Pairs -- 1.2.8.2 The Fuoss Treatment -- 1.2.9 Ion Dynamics -- 1.2.9.1 Ionic Mobility and Transport Numbers -- 1.2.9.2 Diffusion -- 1.2.9.3 Fick's Second Law -- 1.2.9.4 Diffusion Statistics -- 1.3 Dynamic Electrochemistry -- 1.3.1 Review of Fundamentals -- 1.3.1.1 Potential -- 1.3.1.2 Potential inside a Good Conductor -- 1.3.1.3 Charge on a Good Conductor -- 1.3.1.4 Force between Charges -- 1.3.1.5 Potential due to an Assembly of Charges -- 1.3.1.6 Potential Difference between Two Phases in Contact (Do) -- 1.3.1.7 The Electrochemical Potential (u) -- 1.3.2 The Electrically Charged Interface or Double Layer -- 1.3.2.1 The Interface -- 1.3.2.2 Ideally Polarized Electrode -- 1.3.2.3 The Helmholtz Model -- 1.3.2.4 Gouy-Chapman or Diffuse Model -- 1.3.2.5 The Stern Model -- 1.3.2.6 The Bockris, Devanathan, and Muller Model -- 1.3.2.7 Calculation of the Capacitance -- 1.3.3 Charge Transfer at the Interface -- 1.3.3.1 Transition State Theory -- 1.3.3.2 Redox Charge-Transfer Reactions -- 1.3.3.3 The Act of Charge Transfer -- 1.3.3.4 The Butler-Volmer Equation -- 1.3.3.5 I in Terms of the Standard Rate Constant (k0).
1.3.3.6 Relation between k0 and I0 -- 1.3.4 Multistep Processes -- 1.3.4.1 The Multistep Butler-Volmer Equation -- 1.3.4.2 Rules for Mechanisms -- 1.3.4.3 Concentration Dependence of I0 -- 1.3.4.4 Charge-Transfer Resistance (Rct) -- 1.3.4.5 Whole Cell Voltages -- 1.3.5 Mass Transport Control -- 1.3.5.1 Diffusion and Migration -- 1.3.5.2 The Limiting Current Density (IL) -- 1.3.5.3 Rotating Disk Electrode -- Further Reading -- 2 General Properties of Electrochemical Capacitors -- 2.1 Introduction -- 2.2 Capacitor Principles -- 2.3 Electrochemical Capacitors -- 2.3.1 Electric Double-Layer Capacitors -- 2.3.1.1 Double-Layer and Porous Materials Models -- 2.3.1.2 EDLC Construction -- 2.3.2 Pseudocapacitive Electrochemical Capacitors -- 2.3.2.1 Electronically Conducting Polymers -- 2.3.2.2 Transition Metal Oxides -- 2.3.2.3 Lithium-Ion Capacitors -- 2.4 Summary -- Acknowledgments -- References -- 3 Electrochemical Techniques -- 3.1 Electrochemical Apparatus -- 3.2 Electrochemical Cell -- 3.3 Electrochemical Interface: Supercapacitors -- 3.4 Most Used Electrochemical Techniques -- 3.4.1 Transient Techniques -- 3.4.1.1 Cyclic Voltammetry -- 3.4.1.2 Galvanostatic Cycling -- 3.4.2 Stationary Technique -- 3.4.2.1 Electrochemical Impedance Spectroscopy -- 3.4.2.2 Supercapacitor Impedance -- References -- 4 Electrical Double-Layer Capacitors and Carbons for EDLCs -- 4.1 Introduction -- 4.2 The Electrical Double Layer -- 4.3 Types of Carbons Used for EDLCs -- 4.3.1 Activated Carbon Powders -- 4.3.2 Activated Carbon Fabrics -- 4.3.3 Carbon Nanotubes -- 4.3.4 Carbon Aerogels -- 4.4 Capacitance versus Pore Size -- 4.5 Evidence of Desolvation of Ions -- 4.6 Performance Limitation: Pore Accessibility or Saturation of Porosity -- 4.6.1 Limitation by Pore Accessibility -- 4.6.2 Limitation of Capacitor Performance by Porosity Saturation.
4.7 Beyond the Double-Layer Capacitance in Microporous Carbons -- 4.7.1 Microporous Carbons in Neat Ionic Liquid Electrolyte -- 4.7.2 Extra Capacitance with Ionic Liquids in Solution -- 4.7.3 Ions Trapping in Pores -- 4.7.4 Intercalation/Insertion of Ions -- 4.8 Conclusions -- References -- 5 Modern Theories of Carbon-Based Electrochemical Capacitors -- 5.1 Introduction -- 5.1.1 Carbon-Based Electrochemial Capacitors -- 5.1.2 Elements of EDLCs -- 5.2 Classical Theories -- 5.2.1 Compact Layer at the Interface -- 5.2.2 Diffuse Layer in the Electrolyte -- 5.2.3 Space Charge Layer in the Electrodes -- 5.3 Recent Developments -- 5.3.1 Post-Helmholtz Models with Surface Curvature Effects -- 5.3.1.1 Models for Endohedral Capacitors -- 5.3.1.2 Models for Hierarchically Porous Carbon Materials -- 5.3.1.3 Models for Exohedral Capacitors -- 5.3.2 EDL Theories Beyond the GCS Model -- 5.3.3 Quantum Capacitance of Graphitic Carbons -- 5.3.4 Molecular Dynamics Simulations -- 5.3.4.1 EDLs in Aqueous Electrolytes -- 5.3.4.2 EDLs in Organic Electrolytes -- 5.3.4.3 EDLs in Room-Temperature ILs -- 5.4 Concluding Remarks -- Acknowledgments -- References -- 6 Electrode Materials with Pseudocapacitive Properties -- 6.1 Introduction -- 6.2 Conducting Polymers in Supercapacitor Application -- 6.3 Metal Oxide/Carbon Composites -- 6.4 Pseudocapacitive Effect of Heteroatoms Present in the Carbon Network -- 6.4.1 Oxygen-Enriched Carbons -- 6.4.2 Nitrogen-Enriched Carbons -- 6.5 Nanoporous Carbons with Electrosorbed Hydrogen -- 6.6 Electrolytic Solutions - a Source of Faradaic Reactions -- 6.7 Conclusions - Profits and Disadvantages of Pseudocapacitive Effects -- References -- 7 Li-Ion-Based Hybrid Supercapacitors in Organic Medium -- 7.1 Introduction -- 7.2 Voltage Limitation of Conventional EDLCs -- 7.3 Hybrid Capacitor Systems -- 7.3.1 Lithium-Ion Capacitor (LIC).
7.3.2 Nanohybrid Capacitor (NHC) -- 7.4 Material Design for NHC -- 7.5 Conclusion -- Abbreviations -- References -- 8 Asymmetric and Hybrid Devices in Aqueous Electrolytes -- 8.1 Introduction -- 8.2 Aqueous Hybrid (Asymmetric) Devices -- 8.2.1 Principles, Requirements, and Limitations -- 8.2.2 Activated Carbon/PbO2 Devices -- 8.2.3 Activated Carbon/Ni(OH)2 Hybrid Devices -- 8.2.4 Aqueous-Based Hybrid Devices Based on Activated Carbon and Conducting Polymers -- 8.3 Aqueous Asymmetric Electrochemical Capacitors -- 8.3.1 Principles, Requirements, and Limitations -- 8.3.2 Activated Carbon/MnO2 Devices -- 8.3.3 Other MnO2-Based Asymmetric or Hybrid Devices -- 8.3.4 Carbon/Carbon Aqueous Asymmetric Devices -- 8.3.5 Carbon/RuO2 Devices -- 8.4 Tantalum Oxide-Ruthenium Oxide Hybrid Capacitors -- 8.5 Perspectives -- References -- 9 EDLCs Based on Solvent-Free Ionic Liquids -- 9.1 Introduction -- 9.2 Carbon Electrode/Ionic Liquid Interface -- 9.3 Ionic Liquids -- 9.4 Carbon Electrodes -- 9.5 Supercapacitors -- 9.6 Concluding Remarks -- Ionic Liquid Codes -- Glossary -- References -- 10 Manufacturing of Industrial Supercapacitors -- 10.1 Introduction -- 10.2 Cell Components -- 10.2.1 Electrode Design and Its Components -- 10.2.1.1 Current Collector -- 10.2.1.2 Activated Carbons for Supercapacitors -- 10.2.1.3 Industrial Activated Carbons for Industrial Supercapacitors -- 10.2.1.4 Particle Size Distribution of Activated Carbons and Its Optimization -- 10.2.1.5 Binders -- 10.2.1.6 Conductive Additives -- 10.2.2 Electrolyte -- 10.2.2.1 Electrolyte Impact on Performance -- 10.2.2.2 Liquid-State Electrolyte and Remaining Problems -- 10.2.2.3 Ionic Liquid Electrolyte -- 10.2.2.4 Solid-State Electrolyte -- 10.2.3 Separator -- 10.2.3.1 Separator Requirements -- 10.2.3.2 Cellulosic Separators and Polymeric Separators -- 10.3 Cell Design -- 10.3.1 Small-Size Components.
10.3.2 Large Cells.
Abstract:
Supercapacitors are a relatively new energy storage system that provides higher energy density than dielectric capacitors and higher power density than batteries. They are particularly suited to applications that require energy pulses during short periods of time, e.g., seconds or tens of seconds. They are recommended for automobiles, tramways, buses, cranes, fork-lifts, wind turbines, electricity load leveling in stationary and transportation systems, etc. Despite the technological maturity of supercapacitors, there is a lack of comprehensive literature on the topic. Many high performance materials have been developed and new scientific concepts have been introduced. Taking into account the commercial interest in these systems and the new scientific and technological developments now is the ideal time to publish this book, capturing all this new knowledge. The book starts by giving an introduction to the general principles of electrochemistry, the properties of electrochemical capacitors, and electrochemical characterization techniques. Electrical double layer capacitors and pseudocapacitors are then discussed, followed by the various electrolyte systems. Modelling, manufacture of industrial capacitors, constraints, testing, and reliability as well as applications are also covered. 'Supercapacitors - Materials, Systems, and Applications' is part of the series on Materials for Sustainable Energy and Development edited by Prof. G.Q. Max Lu. The series covers advances in materials science and innovation for renewable energy, clean use of fossil energy, and greenhouse gas mitigation and associated environmental technologies.
Local Note:
Electronic reproduction. Ann Arbor, Michigan : ProQuest Ebook Central, 2017. Available via World Wide Web. Access may be limited to ProQuest Ebook Central affiliated libraries.
Genre:
Electronic Access:
Click to View