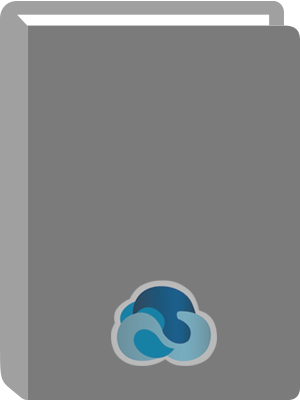
Engineering Materials For Biomedical Applications.
Title:
Engineering Materials For Biomedical Applications.
Author:
Hin, Teoh Swee.
ISBN:
9789812562227
Personal Author:
Physical Description:
1 online resource (350 pages)
Contents:
ENGINEERING MATERIALS FOR BIOMEDICAL APPLICATIONS -- CONTENTS -- Foreword -- Preface -- Acknowledgements -- 1 Introduction to biomaterials engineering and processing - an overview (S. H. Teoh) -- 1.1 Introduction -- 1.2 Requirements of biomaterials -- 1.3 Classification of biomaterials -- 1.4 Mechanical properties of biomaterials -- 1.5 Effects of processing on properties biomaterials -- 1.5.1 Effect of post processing and grain size -- 1.5.2 Effect of molding conditions and irradiation on polymeric wear -- 1.5.3 Effect of composite lamination -- 1.6 Tissue engineering - new wave in biomaterials engineering -- 1.6.1 Need for organ and tissue replacement -- 1.6.2 Limitation of current technologies -- 1.6.3 Platform technology development in tissue engineering -- 1.6.4 Tissue engineering issues and challenges -- 1.7 Conclusions -- References -- 2 Durability of metallic implant materials (M. Sumita and S. H. Teoh) -- 2.1 Introduction -- 2.2 Typical metallic biomaterials -- 2.2.1 Stainless steels -- 2.2.2 Cobalt-chromium alloys -- 2.2.3 Titanium and its alloys -- 2.2.4 Nickel-titanium alloys -- 2.3 Body environment to metallic materials -- 2.4 Life of implanted metallic materials -- A. Degree of damages to implanted metallic materials due to the human body environment -- B. Degree of damages to the human body caused by implanted metallic materials -- 2.5 Corrosion, wear/fretting and fatigue -- 2.5.1 Fatigue testing method -- 2.5.2 Notes for fatigue/fretting fatigue tests -- 2.5.3 Corrosion fatigue -- 2.5.4 Fretting corrosion fatigue -- 2.5.4.1 Fretting fatigue -- 2.5.4.2 Metallic ions release from fretted side -- 2.5.4.3 Fatigue and fretting fatigue strengths at high cycle for typical metallic biomaterials in pseudo-body fluid -- (a) Friction coefficient between the specimen and the pad.
(b) Relative site of the boundary between stick and slip regions at the fretted area of the specimen -- (c) Corrosion pits formed on the fresh metal surface of the fretted area -- (d) Hydrogen generated on the stick regions -- (e) Paring off the micro cracks initiated at the fretted areas -- (f) Temperature rise on the fretted areas in PBS(-) -- 2.5.4.4 Some failures of implants -- 2.6 Toxicity reaction to metallic implants -- 2.7 Metallic biomaterials for the future -- References -- 3 Corrosion of metallic implants (D. J. Blackwood, K. H. W. Seah and S. H. Teoh) -- 3.1 Introduction -- 3.2 Corrosion theory -- 3.2.1 Basic thermodynamics of corrosion -- 3.2.1.1 The Nernst equation -- 3.2.1.2 Standard potentials (E ) and the electrochemical series -- 3.2.1.3 Potential-pH equilibrium diagrams (Pourbaix diagrams) -- 3.2.2 Basic electrochemistry -- 3.2.2.1 Electrode reactions -- 3.2.2.2 Electron transfer -- 3.2.2.3 Kinetics of electron transfer -- 3.2.2.4 Mixed potential theory -- 3.2.2.5 Cathodic reactions -- Oxygen Reduction -- Hydrogen Evolution Reaction -- Definition of Anaerobic Corrosion -- 3.2.2.6 Nature of electrode reactions -- 3.2.3 Passivation -- 3.2.3.1 Electrochemical behavior of active/passive metals -- 3.2.3.2 Nature of passive film -- 3.2.3.3 Influence of cathodic supporting reactions -- 3.3 Types of corrosion -- 3.3.1 General corrosion -- 3.3.2 Localized corrosion -- 3.3.2.1 Pitting corrosion -- 3.3.2.2 Crevice corrosion -- 3.3.2.3 Stress corrosion cracking -- 3.3.2.4 Corrosion fatigue -- 3.3.2.5 Fretting corrosion and mechanical wear -- 3.3.3 Galvanic corrosion (bimetallic corrosion) -- 3.3.4 Selective leaching -- 3.3.5 Intergranular attack -- 3.3.6 Influence of cold-working -- 3.4 Environments encountered in biomedical applications -- 3.4.1 Surgical implants -- 3.4.2 Dental applications.
3.5 Common metals and alloys used in biomedical applications -- 3.5.1 Surgical implants -- 3.5.1.1 Stainless steel -- 3.5.1.2 Cobalt-chromium alloys -- 3.5.1.3 Titanium and titanium alloys -- 3.5.1.4 Porous titanium -- 3.5.1.5 Nickel-titanium alloy -- 3.5.2 Dental materials -- 3.5.2.1 Amalgams -- 3.5.2.2 Rare earth magnets -- 3.6 Detection methods -- 3.7 Corrosion prevention -- 3.7.1 Coatings and surface treatment -- 3.7.2 Quality control -- 3.7.3 Reduce risk of galvanic corrosion -- 3.7.4 Handling/Sterilization/Assembly -- 3.7.5 Education -- 3.8 Case histories -- Case Study A -- Case Study B -- Case Study C -- Case Study D -- Case Study E -- Case Study F -- 3.9 Summary and conclusions -- References -- 4 Surface modification of metallic biomaterials (T. Hanawa) -- 4.1 Surface of metals -- 4.2 Surface oxide film -- 4.2.1 Titanium -- 4.2.2 Titanium alloys -- 4.2.3 Stainless steel -- 4.2.4 Co-Cr-Mo alloy -- 4.2.5 Noble metal alloys -- 4.3 Reconstruction of surface oxide film -- 4.3.1 Titanium -- 4.3.2 Titanium alloys -- 4.3.3 Stainless steel -- 4.3.4 Co-Cr-Mo alloy -- 4.4 Adsorption of proteins -- 4.5 Adhesion of cells -- 4.6 Surface modification -- 4.6.1 Purpose -- 4.6.2 Dry process -- 4.6.3 Hydro-process -- 4.7 Apatite film formation -- 4.7.1 Apatite formation with dry process -- 4.7.2 Apatite formation with hydro-process -- 4.8 Surface-modified layer for bone formation -- 4.8.1 Immersion in alkaline solution and heating -- 4.8.2 Immersion in hydrogen peroxide solution -- 4.8.3 Immersion and hydrothermal treatment in calcium-containing solution -- 4.8.4 Calcium ion implantation -- 4.9 Titanium oxide layer formation -- 4.10 Titanium nitride layer formation -- 4.11 Modification with biomolecules and polymers -- 4.12 Morphological modification -- 4.13 Surface analysis -- 4.14 Future of surface engineering of metallic biomaterials -- References.
5 Biorestorative materials in dentistry (A. U. J. Yap) -- 5.1 Introduction -- 5.2 Ceramics -- 5.2.1 Inorganic salts (dental cements) -- 5.2.2 Crystalline and non-crystalline ceramics -- 5.3 Polymers -- 5.3.1 Rigid polymers -- 5.3.2 Polymer composites -- 5.4 Metals -- 5.4.1 Alloys -- 5.4.2 Intermetallic compounds -- 5.5 Conclusions -- References -- 6 Bioceramics: an introduction (B. Ben-Nissan and G. Pezzotti) -- 6.1 Introduction -- 6.2 General concepts in bioceramics -- 6.3 Bioceramics and production methods -- 6.4 Bioinert ceramics in articulation -- 6.4.1 Alumina ceramics -- 6.4.2 Partially stabilized zirconia (PSZ) -- 6.4.3 New modified zirconia implants -- 6.5 Bioresorbable and bioactive ceramics -- 6.5.1 Calcium phosphates for bone replacement applications -- 6.5.2 Simulated body fluid (SBF) -- 6.5.3 Coralline apatites -- 6.5.4 Calcium phosphate coatings -- 6.5.5 Synthetic bone graft ceramics -- 6.5.6 Bioglasses and glass-ceramics -- 6.6 Nano-bioceramics, composites and hybrids -- 6.6.1 Nanoapatite-polymer fiber composites -- 6.6.2 Bioceramics in in situ radiotherapy and hyperthermia -- 6.6.3 Bone cement composites -- 6.6.4 Biomimetic hybrid composites -- 6.7 Design with bioceramics -- 6.8 Future of bioceramics -- References -- 7 Polymeric hydrogels (J. Li) -- 7.1 Introduction -- 7.2 Definition and classification of hydrogels -- 7.2.1 Definition of hydrogels -- 7.2.2 Classification of hydrogels -- 7.3 Chemical hydrogels and their biomedical applications -- 7.3.1 Copolymerization of monomer with cross-linker -- 7.3.2 Cross-linking of water-soluble polymers -- 7.4 Physical hydrogels and their biomedical applications -- 7.4.1 Natural biopolymer hydrogels -- 7.4.2 Thermo-shrinking hydrogels -- 7.4.3 Amphiphilic triblock copolymer hydrogels -- 7.4.4 Other novel synthetic copolymer physical hydrogels -- 7.4.5 Polyelectrolyte complex hydrogels.
7.4.6 Supramolecular hydrogels formed by cyclodextrins and polymers -- References -- 8 Bioactive ceramic-polymer composites for tissue replacement (M. Wang) -- 8.1 Introduction -- 8.2 Structure and properties of bone -- 8.3 Bioceramics and biopolymers -- 8.3.1 Bioactive ceramics -- 8.3.2 Biocompatible polymers -- 8.4 Hydroxyapatite reinforced polyethylene composites for bone replacement -- 8.4.1 Combining hydroxyapatite and polyethylene for bioactive bone analogues -- 8.4.2 Manufacture of hydroxyapatite/polyethylene composites -- 8.4.3 Structure of hydroxyapatite/polyethylene composites -- 8.4.4 Mechanical properties of hydroxyapatite/polyethylene composites -- 8.4.5 In vitro and in vivo assessments -- 8.4.6 Clinical applications -- 8.4.7 Enhanced hydroxyapatite/polyethylene composites -- 8.5 Other bioceramic-polymer composites for medical applications -- 8.6 Concluding remarks -- Acknowledgements -- References -- 9 Composites in biomedical applications (Z. M. Huang and S. Ramakrishna) -- 9.1 Introduction -- 9.2 Biomedical applications -- 9.2.1 Bone plates -- 9.2.2 Intramedullary nails -- 9.2.3 Spine instrumentation -- 9.2.4 Total hip replacement (THR) -- 9.2.5 Bone grafts -- 9.2.6 Dental materials -- 9.2.7 Prosthetic sockets -- 9.2.8 Tendons and ligaments -- 9.2.9 Vascular grafts -- 9.3 Composite fabrication -- 9.3.1 Filament winding -- 9.3.2 Pultrusion -- 9.3.3 Extrusion -- 9.3.4 Injection molding -- 9.3.5 Compression molding -- 9.3.6 Thermoforming -- 9.3.7 A fabrication example -- 9.4 Mechanics of composites -- 9.4.1 RVE and effective property -- 9.4.2 Moduli of UD composite - rule of mixture approach -- 9.4.3 Strengths of UD composite - bridging model formulae -- 9.4.3.1 Longitudinal tensile strength -- 9.4.3.2 Transverse tensile strength -- 9.4.3.3 In-plane shear strength -- 9.4.4 Example -- Solution -- A) Longitudinal Strength.
B) Transverse Strength.
Abstract:
The success of any implant or medical device depends very much on thebiomaterial used. Synthetic materials (such as metals, polymers andcomposites) have made significant contributions to many establishedmedical devices. The aim of this book is to provide a basicunderstanding on the engineering and processing aspects ofbiomaterials used in medical applications.
Local Note:
Electronic reproduction. Ann Arbor, Michigan : ProQuest Ebook Central, 2017. Available via World Wide Web. Access may be limited to ProQuest Ebook Central affiliated libraries.
Genre:
Electronic Access:
Click to View