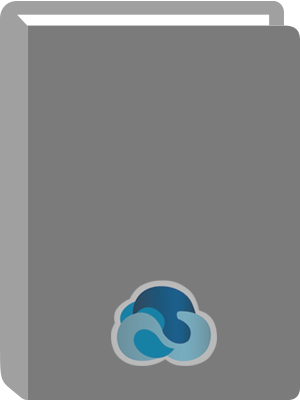
Natural Biodynamics.
Title:
Natural Biodynamics.
Author:
Ivancevic , Vladimir G.
ISBN:
9789812703163
Personal Author:
Physical Description:
1 online resource (1036 pages)
Contents:
Contents -- Preface -- Glossary of Frequently Used Symbols -- 1. Introduction -- 1.1 The Problem of Natural Biodynamics -- 1.2 A Brief History of Biodynamics -- 1.3 Mechanical Basis of Biodynamics -- 1.3.1 Natural Galilei Group -- 1.3.2 Newtonian Equations of Motion -- 1.3.3 Calculus of Variations -- 1.3.4 Lagrangian Equations of Motion -- 1.3.5 Hamiltonian Equations of Motion -- 1.3.6 Lagrangian Flows on Biodynamic Manifolds -- 1.4 Conservative versus Dissipative Hamiltonian Dynamics -- 1.4.1 Dissipative Systems -- 1.4.2 Thermodynamic Equilibrium -- 1.4.3 Nonlinearity -- 1.4.4 The Second Law of Thermodynamics -- 1.4.5 Geometry of Phase Space -- 1.5 Neural Basis of Biodynamics -- 2. Natural Language of Biodynamics -- 2.1 Categorical Metalanguage -- 2.1.1 Preliminaries from Calculus, Algebra and Topology -- 2.1.1.1 Notes From Calculus -- 2.1.1.2 Notes from Set Theory -- 2.1.1.3 Notes from General Topology -- 2.1.1.4 Commutative Diagrams -- 2.1.1.5 Groups and Related Algebraic Structures -- 2.1.2 Categories -- 2.1.3 Functors -- 2.1.4 Natural Transformations -- 2.1.5 Limits and Colimits -- 2.1.6 The Adjunction -- 2.1.7 n-Categories -- 2.1.7.1 Generalization to 'Big' n-Categories -- 2.1.7.2 Topological Structure of n-Categories -- 2.1.8 Algebra in Abelian Categories -- 2.1.9 Fundamental Biodynamic Adjunction -- 2.2 The Basics of Dynamics -- 2.2.1 Ordinary Differential Equations -- 2.2.2 Linear Autonomous Dynamics -- 2.2.2.1 The Flow of a Linear ODE -- 2.2.2.2 Canonical Linear Flows in R2 -- 2.2.2.3 Topological Equivalence -- 2.3 Chaos and Synergetics in Biodynamics -- 2.3.1 Prototype of Chaotic and Synergetic Systems -- 2.3.2 Chaotic Systems and Biomorphs -- 2.3.2.1 Simulation Examples: Chaotic Systems -- 2.3.2.2 Simulation Examples: Biomorphic Systems -- 2.3.3 Controlling Chaos within the Chaos Theory -- 2.3.3.1 Exploiting Critical Sensitivity.
2.3.3.2 Lyapunov exponents and KY-dimension -- 2.3.3.3 Kolmogorov-Sinai entropy -- 2.3.3.4 Chaos Control by Ott, Grebogi and Yorke -- 2.3.3.5 Floquet Stability Analysis and OGY Control -- 2.3.3.6 Jerk Functions of Simple Chaotic Flows -- 2.3.4 The Basic Hamiltonian Model of Biodynamics -- 2.3.5 The Basics of Haken's Synergetics -- 2.3.5.1 Phase Transitions -- 2.3.5.2 Mezoscopic Derivation of Order Parameters -- 2.3.6 Macro-Synergetic Control of Biodynamics -- 3. Natural Geometry of Biodynamics -- 3.1 Motivation for Geometry in Biodynamics -- 3.2 Biodynamic Manifold M -- 3.2.1 Definition of the Manifold M -- 3.2.2 Smooth Maps Between Manifolds -- 3.3 Biodynamic Bundles -- 3.3.1 The Tangent Bundle of the Manifold M -- 3.3.2 The Cotangent Bundle of the Manifold M -- 3.4 Sections of Biodynamic Bundles -- 3.4.1 Biodynamic Evolution and Flow -- 3.4.2 Vector-Fields and Their Flows -- 3.4.2.1 Vector-Fields on M -- 3.4.2.2 Integral Curves as Biodynamic Trajectories -- 3.4.2.3 Biodynamic Flows on M -- 3.4.2.4 Categories of ODES -- 3.4.3 Differential Forms on M -- 3.4.3.1 1-Forms on M -- 3.4.3.2 &Forms on M -- 3.4.3.3 Exterior Differential Systems -- 3.4.3.4 Exterior Derivative on M -- 3.4.3.5 De Rham Complex and Homotopy Operators -- 3.4.3.6 Stokes Theorem and De Rham Cohomology -- 3.4.3.7 Euler-Poincare Characteristics of M -- 3.4.3.8 Duality of Chains and Forms on M -- 3.4.3.9 Other Exterior Operators on M -- 3.4.4 Geometry of Nonlinear Dynamics -- 3.5 Lie Categories in Biodynamics -- 3.5.1 Lie Derivative in Biodynamics -- 3.5.1.1 Lie Derivative on Functions -- 3.5.1.2 Lie Derivative of Vector Fields -- 3.5.1.3 Derivative of the Evolution Operator -- 3.5.1.4 Lie Derivative of Differential Forms -- 3.5.1.5 Lie Derivative of Various Tensor Fields -- 3.5.1.6 Lie Algebras -- 3.5.2 Lie Groups in Biodynamics -- 3.5.2.1 Lie Groups and Their Lie Algebras.
3.5.2.2 Actions of Lie Groups on M -- 3.5.2.3 Basic Biodynamic Groups -- 3.5.2.4 Groups of Joint Rotations -- 3.5.2.5 Special Euclidean Groups of Joint Motions -- 3.5.3 Group Structure of the Biodynamic Manifold M -- 3.5.3.1 Purely Rotational Biodynamic Manifold -- 3.5.3.2 Reduction of the Rotational Manifold -- 3.5.3.3 The Complete Biodynamic Manifold -- 3.5.3.4 Realistic Human Spine Manifold -- 3.5.4 Lie Symmetries in Biodynamics -- 3.5.4.1 Lie Symmetry Groups -- 3.5.4.2 Prolongations -- 3.5.4.3 Special Biodynamic Equations -- 3.6 Riemannian Geometry in Biodynamics -- 3.6.1 Local Riemannian Geometry on M -- 3.6.1.1 Riemannian Metric on M -- 3.6.1.2 Geodesics on M -- 3.6.1.3 Riemannian Curvature on M -- 3.6.2 Global Riemannian Geometry on M -- 3.6.2.1 The Second Variation Formula -- 3.6.2.2 Gauss-Bonnet Formula -- 3.6.2.3 Ricci Flow on M -- 3.6.2.4 Structure Equations on M -- 3.6.2.5 Basics of Morse Theory -- 3.6.2.6 Basics of (Co)Bordism Theory -- 3.7 Symplectic Geometry in Biodynamics -- 3.7.1 Symplectic Algebra -- 3.7.2 Symplectic Geometry on M -- 3.8 Impulse Biodynamics and Synthetic Geometry -- 3.8.1 Delta Spikes -- 3.8.2 Kick Dynamics -- 3.8.2.1 Deterministic Delayed Kicks -- 3.8.2.2 Random Kicks and Langevin Equation -- 3.8.3 Distributions and Synthetic Differential Geometry -- 3.8.3.1 Distributions -- 3.8.3.2 Synthetic Calculus in Euclidean Spaces -- 3.8.3.3 Spheres and Balls as Distributions -- 3.8.3.4 Stokes Theorem for Unit Sphere -- 3.8.3.5 Time Derivatives of Expanding Spheres -- 3.8.3.6 The Wave Equation -- 3.9 A Quick Look at Modern Geometrodynamics -- 3.9.1 Einstein Equations -- 3.9.2 n-Categories in Physics -- 3.9.3 Quantum Geometry Framework -- 3.10 3D Modelling and Animation in Biodynamics -- 3.10.1 Essentials of Human Animation -- 3.10.1.1 Motion CaptureBased Human Animation -- 3.10.1.2 Virtual Muscular Dynamics in 3D-Graphics.
3.10.2 Curves and Surfaces in Geometric Modelling -- 3.10.2.1 Power Basis Form of a Curve -- 3.10.2.2 Bezier Curves -- 3.10.2.3 Rational Bezier Curves -- 3.10.3 B-Spline Basis Functions -- 3.10.3.1 DeBoor-Cox Recursive Definition -- 3.10.3.2 Derivatives of B-Spline Basis Functions -- 3.10.4 B-Spline Curves and Surfaces in Geometric Modelling -- 3.10.4.1 Definition of B-Spline Curves -- 3.10.4.2 Properties of B-Spline Curves -- 3.10.4.3 Derivatives of a B-Spline Curve -- 3.10.4.4 Definition of B-Spline Surfaces -- 3.10.4.5 Properties of B-Spline Surfaces -- 3.10.4.6 Derivatives of a B-Spline Surface -- 3.10.5 NURBS Curves and Surfaces -- 3.10.5.1 Definition of NURBS Curves -- 3.10.5.2 Properties of NURBS Curves -- 3.10.5.3 Definition of NURBS Surfaces -- 3.10.5.4 Properties of NURBS Surfaces -- 3.11 Kinematics of Biomechanical Chains -- 3.11.1 3D Transformation Matrix -- 3.11.2 A Multilink Kinematic Chain -- 3.11.3 CNS Representation of the Body Posture -- 3.11.4 Transformation Matrix Used in Computer Graphics -- 4. Natural Mechanics of Biodynamics -- 4.1 Lagrangian Formalism in Biodynamics -- 4.2 Hamiltonian Formalism in Biodynamics -- 4.2.1 Nonlinear Dynamics in Hamiltonian Form -- 4.2.1.1 Real 1-DOF Hamiltonian Dynamics -- 4.2.1.2 Complex One-DOF Hamiltonian Dynamics -- 4.2.1.3 Library of Basic Hamiltonian Systems -- 4.2.1.4 n-DOF Hamiltonian Dynamics -- 4.2.2 Hamiltonian Geometry in Biodynamics -- 4.2.3 Hamilton-Poisson Geometry in Biodynamics -- 4.2.3.1 Hamilton-Poisson Biodynamic Systems -- 4.2.4 Completely Integrable Hamiltonian Systems -- 4.2.4.1 Liouville Theorem -- 4.2.4.2 Action-Angle Variables -- 4.2.5 Ergodicity -- 4.2.5.1 Ergodicity in Hamiltonian Systems -- 4.2.5.2 Dynamical Systems and Hyperbolicity -- 4.2.5.3 Ergodic Theory and Nontrivial Recurrence -- 4.2.5.4 Nonuniformly Hyperbolic Trajectories.
4.2.5.5 Systems with Nonzero Lyapunov Exponents -- 4.3 Quantum Formalism in Nano-Biodynamics -- 4.3.1 Quantum Mechanics in Biological Matter -- 4.3.2 Dirac's Canonical Quantization -- 4.3.2.1 Quantum States and Operators -- 4.3.2.2 Quantum Pictures -- 4.3.2.3 Spectrum of a Quantum Operator -- 4.3.2.4 General Representation Model -- 4.3.2.5 Direct Product Space -- 4.3.2.6 State-Space for n Quantum Particles -- 4.3.2.7 Quantum Measurement and Penrose Paradox -- 4.3.3 The Problem of Quantum Measurement and Entropy -- 4.3.3.1 The Classical Apparatus -- 4.3.3.2 Quantum Object -- 4.3.3.3 Adiabatic Measurement Lagrangians -- 4.3.3.4 The Stern-Gerlach Experiment -- 4.3.3.5 Work and Heat -- 4.3.3.6 Statistical Thermodynamics -- 4.3.3.7 Friction in a Macroscopic Apparatus -- 4.3.3.8 Low Velocity Projective Measurements -- 4.3.3.9 Information and Entropy -- 4.3.4 Von Neumann's Density Matrix Quantization -- 4.3.4.1 Dissipative Quantum Formalism -- 4.3.5 Geometric Quantization -- 4.3.5.1 Motivation -- 4.3.5.2 Geometric Prequantization -- 4.4 Variational Formalism in Biodynamics -- 4.4.1 Biodynamic Action Functional -- 4.4.2 Lagrangian Action -- 4.4.3 Hamiltonian Action -- 4.4.4 Noether Theorem -- 4.4.5 Hamiltonian-Action Formulation of Biodynamics -- 4.4.6 Feynman Quantum Action -- 4.5 Nonholonomic Biodynamics -- 4.5.1 Lagrangian Approach -- 4.5.2 Hamiltonian Approach -- 4.5.3 Biodynamic Example: Bicycle Dynamics -- 4.6 Stochastic Formalism in Biodynamics -- 4.6.1 Markov Stochastic Processes -- 4.6.2 Statistical Mechanics of Oscillator Chains -- 4.7 Muscular Excitation-Contraction Dynamics -- 4.7.1 Human Musculo-Skeletal System -- 4.7.1.1 Human Skeleton -- 4.7.1.2 Human Joints -- 4.7.1.3 Human Muscular System -- 4.7.1.4 Human Energy Flow -- 4.7.1.5 Equivalent Muscular Actuator -- 4.7.2 Molecular Muscular Dynamics -- 4.7.3 Mezoscopic Muscular Dynamics.
4.7.3.1 Myocybernetics.
Abstract:
This comprehensive volume is a graduate-level text in human biodynamics, written in the unified categorical language of modern differential geometry and topology. Combining mathematics, physics and robotics with human physiology, this is the first book that describes all levels of human biodynamics, from musculo-skeletal mechanics to the higher brain functions. The book develops and uses a variety of research methods, ranging from chaos theory and Hakenâs synergetics, through quantum mechanics, to nonlinear control and artificial intelligence, to provide the means to understand, predict and control the behavior of human-like systems in their full neuro-musculo-skeletal complexity. The applications of this unique scientific methodology range from prediction of human neuro-musculo-skeletal injuries to brain-like control of humanoid robots.
Local Note:
Electronic reproduction. Ann Arbor, Michigan : ProQuest Ebook Central, 2017. Available via World Wide Web. Access may be limited to ProQuest Ebook Central affiliated libraries.
Genre:
Added Author:
Electronic Access:
Click to View