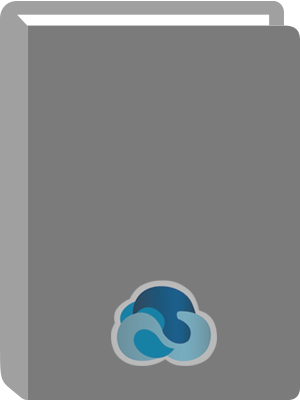
Advances in Chemical Physics, Quantum Information and Computation for Chemistry.
Title:
Advances in Chemical Physics, Quantum Information and Computation for Chemistry.
Author:
Kais, Sabre.
ISBN:
9781118742600
Personal Author:
Edition:
1st ed.
Physical Description:
1 online resource (663 pages)
Series:
Advances in Chemical Physics Ser. ; v.154
Advances in Chemical Physics Ser.
Contents:
Quantum Information and Computation for Chemistry -- Contributors to Volume 154 -- Foreword -- Preface to The Series -- Contents -- Introduction to Quantum Information and Computation for Chemistry -- I. Introduction -- A. Qubits and Gates -- B. Circuits and Algorithms -- C. Teleportation -- II. Quantum Simulation -- A. Introduction -- B. Phase Estimation Algorithm -- 1. General Formulation -- 2. Implementation of Unitary Transformation U -- 3. Group Leaders Optimization Algorithm -- 4. Numerical Example -- 5. Simulation of the Water Molecule -- III. Algorithm for Solving Linear Systems Ax = b -- A. General Formulation -- B. Numerical Example -- IV. Adiabatic Quantum Computing -- A. Hamiltonians of n-Particle Systems -- B. The Model of Adiabatic Computation -- C. Hamiltonian Gadgets -- V. Topological Quantum Computing -- A. Anyons -- B. Non-Abelian Braid Groups -- C. Topological Phase of Matter -- D. Quantum Computation Using Anyons -- VI. Entanglement -- VII. Decoherence -- VIII. Major Challenges and Opportunities -- References -- Back to The Future: A Roadmap for Quantum Simulation from Vintage Quantum Chemistry -- I. Introduction -- II. Quantum Computing -- A. Phase Estimation -- B. Time Evolution and the Cartan Decomposition -- III. Quantum Chemistry: The CI Method -- A. Second Quantization: Direct Mapping -- B. FCI: Compact Mapping -- IV. A Selection of Historical Calculations in Quantum Chemistry -- A. The 1930s and 1940s -- B. The 1950s -- C. The 1960s -- V. Boys's 1950 Calculation for Be -- VI. Conclusions -- References -- Introduction to Quantum Algorithms for Physics and Chemistry -- I. Introduction -- A. Quantum Computational Complexity and Chemistry -- 1. An Exponential Wall for Many-Body Problems -- 2. Computational Complexity of Quantum Simulation -- B. Basic Quantum Algorithms for Digital Quantum Simulation.
1. Quantum Fourier Transform -- 2. Phase Estimation Algorithm -- II. Digital Quantum Simulation -- A. Overview -- B. Simulation of Time Evolution -- 1. Suzuki-Trotter Formulas -- 2. First-Quantized Representation -- 3. Second-Quantized Representation -- 4. Open-System Dynamics -- C. State Preparation -- 1. Preparing Ground States -- 2. Preparing Thermal States Using Quantum Metropolis -- 3. Preparing Thermal States with Perturbative Updates -- D. Algorithmic Quantum Cooling -- 1. Basic Idea of the Quantum Cooling Method -- 2. Connection with Heat-Bath Algorithmic Cooling -- III. Special Topics -- A. Adiabatic Nondestructive Measurements -- B. TDDFT and Quantum Simulation -- IV. Conclusion and Outlook -- References -- Quantum Computing Approach to Nonrelativistic and Relativistic Molecular Energy Calculations -- I. Overview -- II. Quantum Computing Background -- A. Quantum Fourier Transform -- B. Semiclassical Approach to Quantum Fourier Transform -- C. Phase Estimation Algorithm -- D. Iterative Phase Estimation Algorithm -- III. Quantum Full Configuration Interaction Method -- A. Mapping of Quantum Chemical Wave Functions onto Quantum Register -- B. Initial States for the Algorithm -- 1. Adiabatic State Preparation -- C. Controlled "Time Propagation" -- 1. Decomposition of Unitary Propagator to Elementary Quantum Gates -- IV. Application to Nonrelativistic Molecular Hamiltonians -- A. Example of CH2 Molecule -- V. Extension to Relativistic Molecular Hamiltonians -- A. Example of SbH Molecule -- VI. Conclusions -- References -- Density Functional Theory and Quantum Computation -- I. Introduction -- II. Qubit/Fermion Transformation -- III. NP-Complete Problem MAXCUT -- IV. GS-DFT -- V. TD-DFT -- VI. Minimum Gap -- VII. Discussion -- References -- Quantum Algorithms for Continuous Problems and Their Applications -- I. Introduction.
II. The Model of Computation -- A. Quantum Queries -- B. Quantum Algorithms -- III. Applications -- A. Integration -- B. Path Integration -- C. Approximation -- D. Ordinary Differential Equations -- E. Partial Differential Equations -- F. Optimization -- G. Gradient Estimation -- H. Simulation -- I. Eigenvalue Estimation -- J. Linear Systems -- References -- Analytic Time Evolution, Random Phase Approximation, and Green Functions for Matrix Product States -- I. Introduction -- II. Stationary States -- III. Time Evolution and Equations of Motion -- IV. Random Phase Approximation -- V. Green Functions and Correlations -- VI. Conclusion -- References -- Few-Qubit Magnetic Resonance Quantum Information Processors: Simulating Chemistry and Physics -- I. Nuclear Magnetic Resonance QIP -- A. Introduction -- B. Quantum Algorithms for Chemistry -- 1. Digital Quantum Simulation -- 2. Adiabatic Quantum Simulation -- C. NMR and the DiVincenzo Criteria -- 1. Scalability with Well-Characterized Qubits: Spin-1/2 Nuclei -- 2. Initialization: The Pseudopure State -- 3. A Universal Set of Quantum Gates: RF Pulses and Spin Coupling -- 4. Measurement: Free Induction Decay -- 5. Noise and Decoherence: T1/T2 versus Coupling Strength -- II. Quantum Control in Magnetic Resonance QIP -- A. Advances in Pulse Engineering -- B. Advances in Dynamical Decoupling -- C. Control in the Electron-Nuclear System -- 1. Indirect Control via the Anisotropic Hyperfine Interaction -- 2. Dynamic Nuclear Polarization and Algorithmic Cooling -- 3. Spin Buses and Parallel Information Transfer -- III. NMR QIP for Chemistry -- A. Recent Experiments in NMR Quantum Simulation -- 1. Simulation of Burgers' Equation -- 2. Simulation of the Fano-Anderson Model -- 3. Simulation of Frustrated Magnetism -- IV. Prospects for Engineered Spin-Based QIPs -- References.
Photonic Toolbox for Quantum Simulation -- I. Introduction -- II. Toolbox of Photonic Quantum Simulator -- A. Entangled Photon-Pair Source -- B. Encoding and Processing Quantum Information on Single Photon -- C. Photon-Photon Interaction via Measurement -- III. Quantum Chemistry on a Photonic Quantum Computer -- IV. Conclusions -- References -- Progress in Compensating Pulse Sequences for Quantum Computation -- I. Introduction -- II. Coherent Control Over Spin Systems -- A. Errors in Quantum Control -- B. NMR Spectroscopy as a Model Control System -- 1. Error Models -- C. Binary Operations on Unitary Operators -- 1. Hilbert-Schmidt Inner Product -- 2. Fidelity -- III. Group Theoretic Techniques for Sequence Design -- A. Lie Groups and Algebras -- 1. The Spinor Rotation Group SU(2) -- B. Baker-Campbell-Hausdorff and Magnus Formulas -- 1. The Magnus Expansion -- 2. A Method for Studying Compensation Sequences -- C. Decompositions and Approximation Methods -- 1. Basic Building Operations -- 2. Euler Decomposition -- 3. Cartan Decomposition -- IV. Composite Pulse Sequences on SU(2) -- A. Solovay-Kitaev Sequences -- 1. Narrowband Behavior -- 2. Broadband Behavior -- 3. Generalization to Arbitrary Gates in SU(2) -- 4. Arbitrarily Accurate SK Sequences -- B. Wimperis/Trotter-Suzuki Sequences -- 1. Narrowband Behavior -- 2. Broadband Behavior -- 3. Passband Behavior -- 4. Arbitrarily Accurate Trotter-Suzuki Sequences -- C. CORPSE -- 1. Arbitrarily Accurate CORPSE -- 2. Concatenated CORPSE: Correcting Simultaneous Errors -- D. Shaped Pulse Sequences -- V. Composite Pulse Sequences on Other Groups -- A. Compensated Two-Qubit Operations -- 1. Cartan Decomposition of Two-Qubit Gates -- 2. Operations Based on the Ising Interaction -- 3. Extension to SU(2n) -- VI. Conclusions and Perspectives -- References.
Review of Decoherence-Free Subspaces, Noiseless Subsystems, and Dynamical Decoupling -- I. Introduction -- II. Decoherence-Free Subspaces -- A. A Classical Example -- B. Collective Dephasing DFS -- C. Decoherence-Free Subspaces in the Kraus OSR -- D. Hamiltonian DFS -- E. Deutsch's Algorithm -- F. Deutsch's Algorithm with Decoherence -- III. Collective Dephasing -- A. The Model -- B. The DFS -- C. Universal Encoded Quantum Computation -- IV. Collective Decoherence and Decoherence-Free Subspaces -- A. One Physical Qubit -- B. Two Physical Qubits -- C. Three Physical Qubits -- D. Generalization to N Physical Qubits -- E. Higher Dimensions and Encoding Rate -- F. Logical Operations on the DFS of Four Qubits -- V. Noiseless/Decoherence-Free Subsystems -- A. Representation Theory of Matrix Algebras -- B. Computation Over a NS -- C. Example: Collective Decoherence Revisited -- 1. General Structure -- 2. The Three-Qubit Code for Collective Decoherence -- 3. Computation Over the Three-Qubit Code -- VI. Dynamical Decoupling -- A. Decoupling Single-Qubit Pure Dephasing -- 1. The Ideal Pulse Case -- 2. The Real Pulse Case -- B. Decoupling Single-Qubit General Decoherence -- VII. Dynamical Decoupling as Symmetrization -- VIII. Combining Dynamical Decoupling with DFS -- A. Dephasing on Two Qubits: A Hybrid DFS-DD Approach -- B. General Decoherence on Two Qubits: A Hybrid DFS-DD Approach -- IX. Concatenated Dynamical Decoupling: Removing Errors of Higher Order in Time -- X. Dynamical Decoupling and Representation Theory -- A. Information Storage and Computation Under DD -- B. Examples -- 1. Example 1: G = (SU(2))N -- 2. Example 2: G = Collective SU(2) -- 3. Example 3: G = Sn -- 4. Example 4: Linear System-Bath Coupling -- XI. Conclusions -- References -- Functional Subsystems and Strong Correlation in Photosynthetic Light Harvesting -- I. Introduction.
II. Effect of Strong Electron Correlation.
Abstract:
SABRE KAIS received his PhD in Chemical Physics from the Hebrew University in 1989. Since 2002, he has been a full professor of Chemical Physics at Purdue University. He has courtesy professorship appointments at both the Department of Computer Science and the Department of Physics at Purdue, is a member of Qatar Environment and Energy Research Institute, and is External Professor at Santa Fe Institute. Recently, with his colleagues, he established a new center, Quantum Information for Quantum Chemistry (QIQC). STUART A. RICE received his master's degree and doctorate from Harvard University and was a junior fellow at Harvard for two years before joining the faculty of The University of Chicago in 1957, where he is currently the Frank P. Hixon Distinguished Service Professor Emeritus. AARON R. DINNER received his bachelor's degree and doctorate from Harvard University, after which he conducted postdoctoral research at the University of Oxford and the University of California, Berkeley. He joined the faculty at The University of Chicago in 2003.
Local Note:
Electronic reproduction. Ann Arbor, Michigan : ProQuest Ebook Central, 2017. Available via World Wide Web. Access may be limited to ProQuest Ebook Central affiliated libraries.
Genre:
Electronic Access:
Click to View