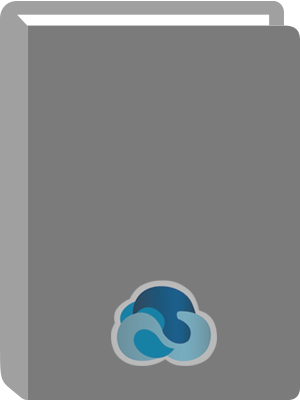
Microstructural Design of Advanced Engineering Materials.
Title:
Microstructural Design of Advanced Engineering Materials.
Author:
Molodov, Dmitri A.
ISBN:
9783527652846
Personal Author:
Edition:
1st ed.
Physical Description:
1 online resource (531 pages)
Contents:
Microstructural Design of Advanced Engineering Materials -- Contents -- Preface -- List of Contributors -- Part I: Materials Modeling and Simulation: Crystal Plasticity, Deformation, and Recrystallization -- 1 Through-Process Modeling of Materials Fabrication: Philosophy, Current State, and Future Directions -- 1.1 Introduction -- 1.2 Microstructure Evolution -- 1.3 Microstructural Processes -- 1.4 Through-Process Modeling -- 1.5 Future Directions -- References -- 2 Application of the Generalized Schmid Law in Multiscale Models: Opportunities and Limitations -- 2.1 Introduction -- 2.2 Crystal Plasticity -- 2.2.1 Generalized Schmid Law -- 2.2.2 Calculation of Slip Rates, Lattice Rotation, and Stress from a Prescribed Deformation -- 2.2.3 Taylor Factor -- 2.3 Polycrystal Plasticity Models for Single-Phase Materials -- 2.3.1 Sachs Model -- 2.3.2 Taylor Theory -- 2.3.3 Relaxed Constraints Taylor Theory -- 2.3.4 Grain Interaction Models -- 2.4 Plastic Anisotropy of Polycrystalline Materials -- 2.5 Experimental Validation -- 2.5.1 Prediction of Rolling Textures -- 2.5.2 Prediction of Cup Drawing Textures -- 2.6 Conclusions -- References -- 3 Crystal Plasticity Modeling -- 3.1 Introduction -- 3.2 Fundamentals -- 3.2.1 Constitutive Models -- 3.2.1.1 Dislocation Slip -- 3.2.1.2 Displacive Transformations -- 3.2.2 Homogenization -- 3.2.3 Boundary Value Solvers -- 3.3 Application Examples -- 3.3.1 Texture and Anisotropy -- 3.3.1.1 Prediction of Texture Evolution -- 3.3.1.2 Prediction of Earing Behavior -- 3.3.1.3 Optimization of Earing Behavior -- 3.3.2 Effective Material Properties -- 3.3.2.1 Direct Transfer of Microstructures -- 3.3.2.2 Representative Volume Elements -- 3.3.2.3 The Virtual Laboratory -- 3.4 Conclusions and Outlook -- References -- 4 Modeling of Severe Plastic Deformation: Time-Proven Recipes and New Results -- 4.1 Introduction.
4.2 One-Internal Variable Models -- 4.3 Two-Internal Variable Models -- 4.4 Three-Internal Variable Models -- 4.5 Numerical Simulations of SPD Processes -- 4.6 Concluding Remarks -- References -- 5 Plastic Anisotropy in Magnesium Alloys - Phenomena and Modeling -- 5.1 Deformation Modes and Textures -- 5.2 Anisotropy of Stress and Strain -- 5.3 Modeling Anisotropic Stress and Strain -- 5.4 Concluding Remarks -- References -- 6 Application of Stochastic Geometry to Nucleation and Growth Transformations -- 6.1 Introduction -- 6.2 Mathematical Background and Basic Notation -- 6.2.1 Modeling Birth-and-Growth Processes -- 6.2.2 Mean Densities Associated to a Birth-and-Growth Process -- 6.2.3 Causal Cone -- 6.3 Revisiting JMAK -- 6.4 Nucleation in Clusters -- 6.4.1 The Matérn Cluster Process -- 6.4.2 Evaluation of the Integral in Eq. (6.17) -- 6.4.3 Numerical Examples -- 6.4.3.1 Influence of Cluster Radius -- 6.4.3.2 Influence of Number of Nuclei per Cluster -- 6.5 Nucleation on Lower Dimensional Surfaces -- 6.5.1 Derivation of General Expressions for Surface and Bulk Nucleation -- 6.5.1.1 Surface Nucleation -- 6.5.1.2 Bulk Nucleation -- 6.5.2 Numerical Examples -- 6.5.2.1 Surface Nucleation -- 6.5.2.2 Bulk Nucleation -- 6.5.2.3 Simultaneous Bulk and Surface Nucleation -- 6.6 Analytical Expressions for Transformations Nucleated on Random Planes -- 6.6.1 General Results for Nucleation on Random Planes -- 6.6.2 Behavior at the Origin as a Model for the Behavior in an "Unbounded "Specimen -- 6.6.3 Nucleation on Random Parallel Planes Located Within a Specimen of Finite Thickness -- 6.6.4 Nucleation on Random Parallel Planes Located Within an "Unbounded Specimens" -- 6.6.5 Computer Simulation Results -- 6.7 Random Velocity -- 6.7.1 Time-Dependent, Random Velocity -- 6.7.2 Particular Cases -- 6.7.3 Computer Simulation.
6.8 Simultaneous and Sequential Transformations -- 6.8.1 Simultaneous Transformations -- 6.8.2 Sequential Transformations -- 6.8.3 Application to Recrystallization of an IF Steel -- 6.9 Final Remarks -- References -- 7 Implementation of Anisotropic Grain Boundary Properties in Mesoscopic Simulations -- 7.1 Introduction -- 7.2 Overview of Simulation Methods -- 7.3 Anisotropy of Grain Boundaries -- 7.3.1 Energy -- 7.3.2 Mobility -- 7.4 Simulation Approaches -- 7.4.1 Potts Model -- 7.4.2 Cellular Automata -- 7.4.3 Phase Field -- 7.4.4 Cusps in Grain Boundary Energy -- 7.4.5 Level Set -- 7.4.6 Vertex -- 7.4.7 Moving Finite Element -- 7.4.8 Particle Pinning of Boundaries -- 7.5 Summary -- References -- Part II: Interfacial Phenomena and their Role in Microstructure Control -- 8 Grain Boundary Junctions: Their Effect on Interfacial Phenomena -- 8.1 Introduction -- 8.2 Experimental Measurement of Grain Boundary Triple Line Energy -- 8.3 Impact of Triple Line Tension on the Thermodynamics and Kinetics in Solids -- 8.3.1 Grain Boundary Triple Line Contribution to the Driving Force for Grain Growth -- 8.3.2 Effect of the Triple Junction Line Tension on the Zener Force -- 8.3.3 Effect of Triple Junction Line Tension on the Gibbs-Thompson Relation -- 8.4 Why do Crystalline Nanoparticles Agglomerate with Low Misorientations? -- 8.5 Concluding Remarks -- References -- 9 Plastic Deformation by Grain Boundary Motion: Experiments and Simulations -- 9.1 Introduction -- 9.2 What is the Coupled Grain Boundary Motion? -- 9.3 Computer Simulation Methodology -- 9.4 Experimental Methodology -- 9.5 Multiplicity of Coupling Factors -- 9.6 Dynamics of Coupled GB Motion -- 9.7 Coupled Motion of Asymmetrical Grain Boundaries -- 9.8 Coupled Grain Boundary Motion and Grain Rotation -- 9.9 Concluding Remarks -- References.
10 Grain Boundary Migration Induced by a Magnetic Field: Fundamentals and Implications for Microstructure Evolution -- 10.1 Introduction -- 10.2 Driving Forces for Grain Boundary Migration -- 10.3 Magnetically Driven Grain Boundary Motion in Bicrystals -- 10.3.1 Specimens and Applied Methods to Measure Grain Boundary Migration -- 10.3.2 Measurements of Absolute Grain Boundary Mobility -- 10.3.3 Misorientation Dependence of Grain Boundary Mobility -- 10.3.4 Effect of Boundary Plane Inclination on Tilt Boundary Mobility -- 10.4 Selective Grain Growth in Locally Deformed Zn Single Crystals under a Magnetic Driving Force -- 10.5 Impact of a Magnetic Driving Force on Texture and Grain Structure Development in Magnetically Anisotropic Polycrystals -- 10.5.1 Texture Evolution during Grain Growth -- 10.5.2 Microstructure Evolution and Growth Kinetics -- 10.6 Magnetic Field Influence on Texture and Microstructure Evolution in Polycrystals Due to Enhanced Grain Boundary Motion -- 10.7 Concluding Remarks -- References -- 11 Interface Segregation in Advanced Steels Studied at theAtomic Scale -- 11.1 Motivation for Analyzing Grain and Phase Boundaries in High-Strength Steels -- 11.2 Theory of Equilibrium Grain Boundary Segregation -- 11.2.1 Gibbs Adsorption Isotherm Applied to Grain Boundaries -- 11.2.2 Langmuir-McLean Isotherm Equations for Grain Boundary Segregation -- 11.2.3 Phase-Field Modeling of Grain Boundary Segregation and Phase Transformation at Grain Boundaries -- 11.2.4 Interface Complexions at Grain Boundaries -- 11.3 Atom Probe Tomography and Correlated Electron Microscopy on Interfaces in Steels -- 11.4 Atomic-Scale Experimental Observation of Grain Boundary Segregation in the Ferrite Phase of Pearlitic Steel -- 11.5 Phase Transformation and Nucleation on Chemically Decorated Grain Boundaries.
11.5.1 Introduction to Phase Transformation at Grain Boundaries -- 11.5.2 Grain Boundary Segregation and Associated Local Phase Transformation in Martensitic Fe-C Steels -- 11.6 Conclusions and Outlook -- References -- 12 Interface Structure-Dependent Grain Growth Behavior in Polycrystals -- 12.1 Introduction -- 12.2 Fundamentals: Equilibrium Shape of the Interface -- 12.2.1 Equilibrium Crystal Shape -- 12.2.2 Equilibrium Boundary Shape -- 12.3 Grain Growth in Solid-Liquid Two-Phase Systems -- 12.3.1 Growth Mechanisms and Kinetics of a Single Crystal in a Liquid -- 12.3.1.1 Diffusion-Controlled Crystal Growth -- 12.3.1.2 Interface Reaction-Controlled Crystal Growth -- 12.3.1.3 Mixed Controlled Growth of a Faceted Crystal -- 12.3.2 Grain Growth Behavior -- 12.3.2.1 Stationary Grain Growth in Systems with Spherical Grains -- 12.3.2.2 Nonstationary Grain Growth in Systems with Faceted Grains -- 12.4 Grain Growth in Solid-State Single-Phase Systems -- 12.4.1 Migration Mechanisms and Kinetics of the Grain Boundary -- 12.4.2 Grain Growth Behavior -- 12.5 Concluding Remarks -- References -- 13 Capillary-Mediated Interface Energy Fields: Deterministic Dendritic Branching -- 13.1 Introduction -- 13.2 Capillary Energy Fields -- 13.2.1 Background -- 13.2.2 Melting Experiments -- 13.2.3 Self-Similar Melting -- 13.2.4 Influence of Capillarity on Melting -- 13.3 Capillarity-Mediated Branching -- 13.3.1 Local Equilibrium -- 13.3.2 Gibbs-Thomson-Herring Interface Potential -- 13.3.3 Tangential Gradients and Fluxes -- 13.3.4 Capillary-Mediated Energy -- 13.4 Branching -- 13.4.1 Stefan Balance -- 13.4.2 Zeros of the Surface Laplacian -- 13.5 Dynamic Solver Results -- 13.6 Conclusions -- References -- Part III: Advanced Experimental Approaches for Microstructure Characterization -- 14 High Angular Resolution EBSD and Its Materials Applications.
14.1 Introduction: Some History of HR-EBSD.
Abstract:
The choice of a material for a certain application is made taking into account its properties. If, for example one would like to produce a table, a hard material is needed to guarantee the stability of the product, but the material should not be too hard so that manufacturing is still as easy as possible - in this simple example wood might be the material of choice. When coming to more advanced applications the required properties are becoming more complex and the manufacturer`s desire is to tailor the properties of the material to fit the needs. To let this dream come true, insights into the microstructure of materials is crucial to finally control the properties of the materials because the microstructure determines its properties. Written by leading scientists in the field of microstructural design of engineering materials, this book focuses on the evolution and behavior of granular microstructures of various advanced materials during plastic deformation and treatment at elevated temperatures. These topics provide essential background and practical information for materials scientists, metallurgists and solid state physicists.
Local Note:
Electronic reproduction. Ann Arbor, Michigan : ProQuest Ebook Central, 2017. Available via World Wide Web. Access may be limited to ProQuest Ebook Central affiliated libraries.
Genre:
Electronic Access:
Click to View