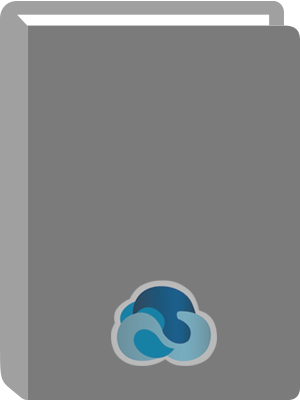
Emerging Nanoelectronic Devices.
Title:
Emerging Nanoelectronic Devices.
Author:
Hutchby, James.
ISBN:
9781118958278
Personal Author:
Edition:
1st ed.
Physical Description:
1 online resource (573 pages)
Contents:
Emerging Nanoelectronic Devices -- Contents -- Preface -- List of Contributors -- Acronyms -- Part One: Introduction -- 1. The Nanoelectronics Roadmap -- 1.1 Introduction -- 1.2 Technology Scaling: Impact and Issues -- 1.3 Technology Scaling: Scaling Limits of Charge-based Devices -- 1.4 The International Technology Roadmap for Semiconductors -- 1.5 ITRS Emerging Research Devices International Technology Working Group -- 1.5.1 ERD Editorial Team -- 1.5.2 Vision and Mission -- 1.5.3 Scope -- 1.6 Guiding Performance Criteria -- 1.6.1 Nanoinformation Processing -- 1.6.2 Nanoelectronic Device Taxonomy -- 1.6.3 Fundamental Guiding Principles - ``Beyond CMOS ́́Information Processing -- 1.6.4 Current Technology Requirements for CMOS Extension and Beyond CMOS Memory and Logic Technologies -- 1.7 Selection of Nanodevices as Technology Entries -- 1.8 Perspectives -- References -- 2. What Constitutes a Nanoswitch? A Perspective -- 2.1 The Search for a Better Switch -- 2.2 Complementary Metal Oxide Semiconductor Switch: Why it Shows Gain -- 2.3 Switch Based on Magnetic Tunnel Junctions: Would it Show Gain? -- 2.3.1 Operation of an MTJ -- 2.3.2 W-R Unit with Electrical Isolation -- 2.3.3 Does This W-R Unit Have Gain? -- 2.4 Giant Spin Hall Effect: A Route to Gain -- 2.4.1 Concatenability -- 2.4.2 Proof of Gain and Directionality -- 2.5 Other Possibilities for Switches with Gain -- 2.5.1 All-spin Logic -- 2.6 What do Alternative Switches Have to Offer? -- 2.6.1 Energy-Delay Product -- 2.6.2 Beyond Boolean Logic -- 2.7 Perspective -- 2.8 Summary -- Acknowledgments -- References -- Part Two: Nanoelectronic Memories -- 3. Memory Technologies: Status and Perspectives -- 3.1 Introduction: Baseline Memory Technologies -- 3.2 Essential Physics of Charge-based Memory -- 3.3 Dynamic Random Access Memory.
3.3.1 Total Energy Required to Create/Maintain the Content of a Memory Cell -- 3.3.2 DRAM Access Time (WRITE or READ) -- 3.3.3 Energy-Space-Time Compromise for DRAM -- 3.4 Flash Memory -- 3.4.1 Store -- 3.4.2 Write -- 3.4.3 Read -- 3.4.4 Energetics of Flash Memory -- 3.5 Static Random Access Memory -- 3.5.1 SRAM Access Time -- 3.5.2 SRAM Scaling -- 3.6 Summary and Perspective -- Appendix: Memory Array Interconnects -- Acknowledgments -- References -- 4. Spin Transfer Torque Random Access Memory -- 4.1 Chapter Overview -- 4.2 Spin Transfer Torque -- 4.2.1 Background of Spin Transfer Torque -- 4.2.2 Experimental Observation of Spin Transfer Torque -- 4.3 STT-RAM Operation -- 4.3.1 Design of STT-RAM Cells -- 4.3.2 Key Parameters for Operation -- 4.4 STT-RAM with Perpendicular Anisotropy -- 4.5 Stack and Material Engineering for Jc Reduction -- 4.5.1 Dual Pinned Structure -- 4.5.2 Nanocurrent Channel Structure Design -- 4.5.3 Electric Field Assisted Switching -- 4.6 Ultra-Fast Switching of MTJs -- 4.7 Spin-Orbit Torques for Memory Application -- 4.8 Current Demonstrations for STT-RAM -- 4.9 Summary and Perspectives -- References -- 5. Phase Change Memory -- 5.1 Introduction -- 5.2 Device Operation -- 5.3 Material Properties -- 5.3.1 Electrical and Phase Transformation Properties -- 5.3.2 Thermal and Mechanical Properties -- 5.4 Device and Material Scaling to the Nanometer Size -- 5.4.1 Materials Scaling Properties -- 5.4.2 Device Scaling Properties -- 5.5 Multi-Bit Operation and 3D Integration -- 5.5.1 Multiple Bits per Element -- 5.5.2 3D Stackable Memory -- 5.6 Applications -- 5.6.1 PCM as a Storage Class Memory -- 5.6.2 PCM as an Electronic Synapse -- 5.7 Future Outlook -- 5.8 Summary -- Acknowledgments -- References -- 6. Ferroelectric FET Memory -- 6.1 Introduction -- 6.2 Ferroelectric FET for Flash Memory Application.
6.2.1 Fe-NAND Flash Memory Operation -- 6.2.2 Nonvolatile Page Buffer -- 6.3 Ferroelectric FET for SRAM Application -- 6.4 System Consideration: SSD System with Fe-NAND Flash Memory -- 6.5 Perspectives and Summary -- References -- 7. Nano-Electro-Mechanical (NEM) Memory Devices -- 7.1 Introduction and Rationale for a Memory Based on NEM Switch -- 7.2 NEM Relay and Capacitor Memories -- 7.3 NEM-FET Memory -- 7.4 Carbon-based NEM Memories -- 7.5 Opportunities and Challenges for NEM Memories -- References -- 8. Redox-based Resistive Memory -- 8.1 Introduction -- 8.2 Physical Fundamentals of Redox Memories -- 8.2.1 Electronic Conduction Mechanisms -- 8.2.2 Ionic Conduction Mechanism -- 8.2.3 Switching Kinetics -- 8.2.4 Generic Switching Properties -- 8.2.5 Modeling Redox Memories for Circuit Simulations -- 8.3 Electrochemical Metallization Memory Cells -- 8.3.1 Physical Switching Mechanism -- 8.3.2 Modeling -- 8.4 Valence Change Memory Cells -- 8.4.1 Physical Switching Mechanism -- 8.4.2 Modeling -- 8.5 Performance -- 8.5.1 Minimum Feature Size -- 8.5.2 Minimum Cell Area -- 8.5.3 Minimum Switching Time -- 8.5.4 Retention Time -- 8.5.5 Write Cycles -- 8.5.6 Multilevel -- 8.6 Summary -- References -- 9. Electronic Effect Resistive Switching Memories -- 9.1 Introduction -- 9.2 Charge Injection and Trapping -- 9.2.1 Charge Trapping Induced Resistive Switching -- 9.2.2 Charge-Trapping Resistive Switching Memory Performance -- 9.3 Mott Transition -- 9.3.1 Resistive Switching Induced by Mott Transition -- 9.3.2 Mott Transition Resistive Switching Memory Performance -- 9.4 Ferroelectric Resistive Switching -- 9.4.1 Ferroelectric Resistive Switching Mechanism -- 9.4.2 Ferroelectric Resistive Switching Memory Performance -- 9.5 Perspectives -- 9.6 Summary -- References -- 10. Macromolecular Memory -- 10.1 Chapter Overview -- 10.2 Macromolecules.
10.2.1 Chemical Structure -- 10.2.2 Memristive Effects in Macromolecular Materials -- 10.2.3 Current State of Macromolecular Memory -- 10.3 Elementary Physical Chemistry of Macromolecular Memory -- 10.3.1 Required Activation Barrier between Stable States -- 10.3.2 Electronic Structure of Macromolecules: Insulators, Semiconductors, Conductors -- 10.3.3 Inducing Changes by Application of Bias Voltage: Electrochemistry -- 10.3.4 Filamentation -- 10.4 Classes of Macromolecular Memory Materials and Their Performance -- 10.4.1 Saturated Macromolecules -- 10.4.2 Macromolecules with π-Conjugation -- 10.5 Perspectives -- 10.6 Summary -- Acknowledgments -- References -- 11. Molecular Transistors -- 11.1 Introduction -- 11.2 Experimental Approaches -- 11.2.1 Fabrication Methods -- 11.2.2 Electronic Transport Fundamentals -- 11.2.3 Molecular Junction Spectroscopies -- 11.3 Molecular Transistors -- 11.3.1 Orbital Gated Transport -- 11.3.2 Coulomb Blockade and Kondo Regimes -- 11.4 Molecular Design -- 11.5 Perspectives -- Acknowledgments -- References -- 12. Memory Select Devices -- 12.1 Introduction -- 12.2 Crossbar Array and Memory Select Devices -- 12.3 Memory Select Device Options -- 12.3.1 Transistors as Memory Select Devices -- 12.3.2 Diodes as Memory Select Devices -- 12.3.3 Volatile Switches as Memory Select Devices -- 12.3.4 Nonlinearity for Device Selection -- 12.4 Challenges of Memory Select Devices -- 12.5 Summary -- References -- 13. Emerging Memory Devices: Assessment and Benchmarking -- 13.1 Introduction -- 13.2 Common Emerging Memory Terminology and Metrics -- 13.3 Redox RAM -- 13.3.1 Electrochemical Metallization Bridge -- 13.3.2 Metal Oxide: Bipolar Filamentary -- 13.3.3 Metal Oxide: Unipolar Filamentary -- 13.3.4 Metal Oxide: Bipolar Nonfilamentary -- 13.4 Emerging Ferroelectric Memories -- 13.4.1 Ferroelectric FET.
13.4.2 Ferroelectric Tunnel Junction -- 13.5 Mott Memory -- 13.6 Macromolecular Memory -- 13.7 Carbon-based Resistive Switching Memory -- 13.7.1 Amorphous Carbon and Diamond-like Carbon -- 13.7.2 Graphene and Graphene Oxide -- 13.7.3 Carbon Nanotubes -- 13.7.4 Research Challenges -- 13.8 Molecular Memory -- 13.9 Assessment and Benchmarking -- 13.9.1 Scaling -- 13.9.2 Performance -- 13.9.3 Reliability -- 13.9.4 CMOS Compatibility and Cost -- 13.9.5 Tradeoffs -- 13.10 Summary and Conclusions -- Acknowledgments -- References -- Part Three: Nanoelectronic Logic and Information Processing -- 14. Re-Invention of FET -- 14.1 Introduction -- 14.2 Historical and Future Trend of MOSFETs -- 14.3 Near-term Solutions -- 14.3.1 High Mobility Channel Transistor -- 14.3.2 Multi-gate Transistor -- 14.3.3 Intrinsic Channel Transistor -- 14.4 Long-term Solutions -- 14.4.1 Energy Efficiency -- 14.4.2 Impact-ionization MOS -- 14.4.3 Tunnel FET -- 14.4.4 Negative Capacitance FET -- 14.4.5 MEMS Switch -- 14.4.6 Mott Transistor -- 14.4.7 Bilayer Pseudo-spin Field-effect Transistor -- 14.5 Summary -- References -- 15. Graphene Electronics -- 15.1 Introduction -- 15.2 Properties of Graphene -- 15.2.1 Bandgap Energy -- 15.2.2 Carrier Transport -- 15.2.3 Heat Transport -- 15.2.4 Contact Resistance -- 15.3 Graphene MOSFETs for Mainstream Logic and RF Applications -- 15.4 Graphene MOSFETs for Nonmainstream Applications -- 15.5 Graphene NonMOSFET Transistors -- 15.6 Perspectives -- 15.6.1 General Remarks -- 15.6.2 Mid-term Perspectives -- 15.6.3 Long-term Future -- Acknowledgment -- References -- 16. Carbon Nanotube Electronics -- 16.1 Carbon Nanotubes - The Ideal Transistor Channel -- 16.1.1 Electronic Structure of a CNT -- 16.1.2 Electron Transport in CNTs -- 16.1.3 The Ideal Transistor -- 16.2 Operation of the CNTFET -- 16.3 Important Aspects of CNTFETs -- 16.3.1 Contacts.
16.3.2 n-Type CNTFETs.
Abstract:
Emerging Nanoelectronic Devices focuses on the future direction of semiconductor and emerging nanoscale device technology. As the dimensional scaling of CMOS approaches its limits, alternate information processing devices and microarchitectures are being explored to sustain increasing functionality at decreasing cost into the indefinite future. This is driving new paradigms of information processing enabled by innovative new devices, circuits, and architectures, necessary to support an increasingly interconnected world through a rapidly evolving internet. This original title provides a fresh perspective on emerging research devices in 26 up to date chapters written by the leading researchers in their respective areas. It supplements and extends the work performed by the Emerging Research Devices working group of the International Technology Roadmap for Semiconductors (ITRS). Key features: Serves as an authoritative tutorial on innovative devices and architectures that populate the dynamic world of "Beyond CMOS" technologies. Provides a realistic assessment of the strengths, weaknesses and key unknowns associated with each technology. Suggests guidelines for the directions of future development of each technology. Emphasizes physical concepts over mathematical development. Provides an essential resource for students, researchers and practicing engineers.
Local Note:
Electronic reproduction. Ann Arbor, Michigan : ProQuest Ebook Central, 2017. Available via World Wide Web. Access may be limited to ProQuest Ebook Central affiliated libraries.
Genre:
Electronic Access:
Click to View