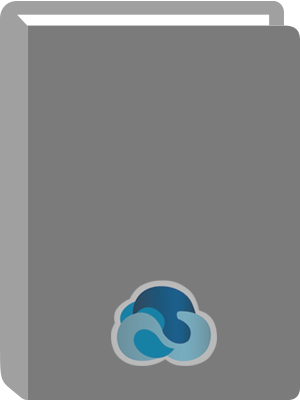
Protein Aggregation in Bacteria : Functional and Structural Properties of Inclusion Bodies in Bacterial Cells.
Title:
Protein Aggregation in Bacteria : Functional and Structural Properties of Inclusion Bodies in Bacterial Cells.
Author:
Doglia, Silvia Maria.
ISBN:
9781118855034
Personal Author:
Edition:
1st ed.
Physical Description:
1 online resource (300 pages)
Series:
Wiley Series in Protein and Peptide Science Ser.
Contents:
Protein Aggregation in Bacteria: Functional and Structural Properties of Inclusion Bodies in Bacterial Cells -- Copyright -- Contents -- Contributors -- Preface -- Introduction to the Wiley Series in Protein and Peptide Science -- 1 Fundamentals of Protein Folding -- 1.1 Folding-misfolding-nonfolding crossroads -- 1.2 Protein folding -- 1.2.1 Protein-Folding Code -- 1.2.2 Protein-Folding Models -- 1.2.3 Polymer Aspects of Protein Folding -- 1.2.4 Different Conformations Seen in Protein Folding -- 1.3 Nonfolding -- 1.3.1 Intrinsically Disordered Proteins and Their Abundance -- 1.3.2 Some Functional Advantages of IDPs -- 1.3.3 Function-Induced Folding of IDPs -- 1.3.4 IDPs and Human Diseases -- 1.3.5 How Does an Amino Acid Sequence Encode Intrinsic Disorder? -- 1.3.6 Polymer Aspects of Nonfolding -- 1.4 Misfolding -- 1.4.1 Molecular Mechanisms of Protein Misfolding -- 1.4.2 Fibrillogenesis of Globular Proteins: Requirement for Partial Unfolding -- 1.4.3 Fibrillogenesis of IDPs: Requirement for Partial Folding -- 1.4.4 Conformational Prerequisites for Amyloidogenesis -- 1.4.5 Multiple Pathways of Protein Misfolding -- 1.4.6 Polymer Aspects of Protein Misfolding -- References -- 2 Recruiting Unfolding Chaperones to Solubilize Misfolded Recombinant Proteins -- 2.1 Introduction -- 2.2 Chemical Chaperones -- 2.3 PPIs and PDIs are folding enzymes -- 2.4 Molecular Chaperones -- 2.5 The small Hsps -- 2.6 Hsp90 -- 2.7 Hsp70/Hsp40 -- 2.8 GroEL Chaperonins -- 2.9 Conclusions -- References -- 3 Osmolytes as Chemical Chaperones to Use in Protein Biotechnology -- 3.1 Introduction -- 3.2 Protein-destabilizing conditions and counteracting mechanisms: shared or independent routes? -- 3.3 Proposed molecular mechanisms for osmolyte activities -- 3.4 Osmolytes and expression of recombinant proteins.
3.5 Biotechnological relevance of osmolytes for preserving purified proteins -- 3.6 Conclusions -- References -- 4 Inclusion Bodies in the Study of Amyloid Aggregation -- 4.1 Introduction -- 4.2 Structure of IBs -- 4.2.1 Amyloid-like Nature of IBs -- 4.2.2 Detection and Characterization of Amyloid Conformations Inside IBs -- 4.3 Formation of IBs -- 4.3.1 In Vivo Formation Kinetics -- 4.3.2 Molecular Determinants of IB Aggregation -- 4.3.3 Sequence Specificity in IB Formation -- 4.4 IBs as the simplest model for in vivo amyloid toxicity -- 4.4.1 The Fitness Cost of Amyloid Aggregation -- 4.4.2 Citotoxicity of Amyloid IBs -- 4.4.3 Infectious Properties of IBs -- 4.5 Using IBs to screen for amyloid inhibitors -- 4.6 Conclusions -- References -- 5 Protein Aggregation in Unicellular Eukaryotes -- 5.1 Introduction -- 5.2 UPR: Unfolded protein response in the ER -- 5.3 Removing persistent misfolded proteins with the proteasome -- 5.4 Lysosomal/vacuolar proteolysis (overload UPS) -- 5.4.1 Autophagy -- 5.4.2 Selective Types of Autophagy -- 5.5 Refolding of protein aggregates in cytosol and nucleus -- 5.6 JUNQ and IPOD -- 5.7 Segregation of aggregates in yeast -- 5.8 Proteins forming nonpathological amyloid-like fibrils in unicellular eukaryotes -- 5.9 Humanized yeast models -- 5.10 Concluding remarks -- Acknowledgments -- References -- 6 Structural Properties of Bacterial Inclusion Bodies -- 6.1 Introduction -- 6.2 Intermediate species in inclusion body formation -- 6.3 Structural characterization of inclusion bodies -- 6.3.1 Composition, Overall Structure, Shape, and Morphology -- 6.3.2 Native-like and Amyloid-like Structures -- 6.4 Appendix: experimental methods Used in IB structural characterization -- References -- 7 Residue-Specific Structural Studies of Inclusion Bodies -- 7.1 Introduction -- 7.2 Molecular structure of amyloid fibrils.
7.2.1 Structure of Peptide Amyloid Fibrils as Determined by X-ray Crystallography -- 7.2.2 Structure of HET-s Amyloid Fibrils as Determined by Solid -State NMR Spectroscopy -- 7.2.3 Cross- β -Sheet Core of HET-s Amyloid Fibrils as Determined by Solution-tate NMR and the H /D-xchange Method -- 7.3 Structural study of inclusion bodies with solution-state NMR and the H/D-exchange method -- 7.3.1 Experimental Mechanism of Solution-State NMR and the H/D-Exchange Method -- 7.3.2 Example: Structural Study of BMP2 (13-74) Inclusion Bodies -- 7.4 Structural study of inclusion bodies with solid-state NMR spectroscopy -- 7.4.1 Structural Study of HET-s(218-289) Inclusion Bodies -- 7.4.2 Structural Study of FHA2 Inclusion Bodies -- 7.5 Summary -- References -- 8 Biomedical Applications of Bacterial Inclusion Bodies -- 8.1 Biology of IB proteins -- 8.2 IB protein quality -- 8.3 IB architecture -- 8.4 IBs as biomaterials -- 8.5 Purification of IBs -- 8.6 IBs in tissue engineering -- 8.7 Slow drug release from bacterial IBs used as Nanopills -- 8.8 Conclusions -- References -- 9 Aggregation of Recombinant Proteins: Understanding Basic Issues to Overcome Production Bottlenecks -- 9.1 Introduction -- 9.2 How do cells react to the overproduction of a recombinant protein? -- 9.2.1 Physiological Responses to Protein Overproduction -- 9.2.2 Stress Response to Protein Overproduction -- 9.2.3 Effects of Protein Overproduction on the Cell Membrane -- 9.3 Structure, composition, and mechanism of deposition of inclusion bodies -- 9.4 From knowledge to application -- 9.4.1 Growth Conditions -- 9.4.2 Coexpression with Chaperones and Folding Modulators -- 9.4.3 Expressivity Tags -- 9.4.4 Protein Engineering as a Tool to Improve Protein Solubility -- 9.4.5 What Have We Learned? Lessons from Directed Evolution -- 9.5 Advantages of using inclusion bodies -- References.
10 Fusion to a Pull-Down Module : Designing Enzymes to Form BioCatalytically Active Insoluble Aggregates -- 10.1 Introduction -- 10.2 A short glance at CLEAs -- 10.3 Pull-down modules and their use in inducing selective protein aggregation -- 10.4 Self-aggregating tags as pull-down modules -- 10.5 Pull-down modules undergoing ordered self-assembly -- 10.6 Auto-encapsulation -- 10.7 Even simpler enzymatically active inclusion bodies derived from recombinant proteins as such -- 10.8 Conclusions -- References -- Index -- Supplemental Images.
Abstract:
SILVIA MARIA DOGLIA, PhD, is Professor of Physics at the University of Milano-Bicocca, Italy. She received her Laurea degree in Physics at the University of Milano. She has been Staff Research Scientist of the Italian National Research Council; Visiting Scientist at the University of Stockholm; Visiting Professor at the Universities of Orléans (Fr) and of Reims (Fr). Her research in Biophysics at the University of Milano-Bicocca is focused on the study of protein folding and aggregation in vitro and in situ. MARINA LOTTI, PhD, is Professor of Biochemistry at the University of Milano-Bicocca, Italy, where she leads the group Protein Engineering and Industrial Enzymology, and is the Head of the Department of Biotechnology and Biosciences. She obtained her PhD degree at the Max-Planck Institute of Molecular Genetics, Berlin, and was a researcher of the Italian National Research Council. Major research topics include the production of recombinant proteins, protein aggregation, cold-active enzymes, and intrinsically disordered proteins.
Local Note:
Electronic reproduction. Ann Arbor, Michigan : ProQuest Ebook Central, 2017. Available via World Wide Web. Access may be limited to ProQuest Ebook Central affiliated libraries.
Subject Term:
Genre:
Added Author:
Electronic Access:
Click to View