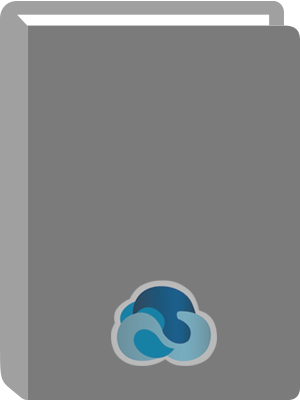
Biomechanical Systems Technology (A 4-Volume Set) : (2) Cardiovascular Systems.
Title:
Biomechanical Systems Technology (A 4-Volume Set) : (2) Cardiovascular Systems.
Author:
Leondes, Cornelius T.
ISBN:
9789812771377
Personal Author:
Physical Description:
1 online resource (278 pages)
Contents:
CONTENTS -- Preface -- Chapter 1 A Simulation Study of Hemodynamic Benefits and Optimal Control of Axial Flow Pump-Based Left Ventricular Assist Device Huiting Qiao, Jing Bai and Ping He -- 1. Introduction -- 2. A Brief Review of Ventricular Assist Devices -- 2.1. General objectives of ventricular assist devices -- 2.2. Classification of ventricular assist devices -- 2.2.1. Pulsatile and nonpulsatile assist devices -- 2.2.2. Parallel and sequential assist devices -- 2.2.3. Axial blood pump and other types of ventricular assist devices -- 2.3. Intra-aortic balloon pump, Hemopump and dynamic aortic valve -- 2.3.1. Intra-aortic balloon pump -- 2.3.2. Hemopump -- 2.3.3. Dynamic aortic valve -- 3. Simulation Study of the Hemopump -- 3.1. Modeling the Hemopump -- 3.1.1. Static model of the pump -- 3.1.2. Dynamic model of the axial flow pump -- 3.1.3. Cardiovascular model including the axial flow pump -- 3.2. Computer simulations and results -- 3.2.1. The pump flow and pressure difference during one cardiac cycle -- 3.2.2. The effects of the pump on various hemodynamic variables -- 3.2.3. The beneficial effects of the axial flow pump on a failing heart -- 4. Optimum Control of the Axial Flow Pump -- 4.1. The objective function for optimal control of the axial flow pump -- 4.1.1. Determine the members of the objective function -- 4.1.2. Establish the membership function -- 4.1.3. Determine the weighting factor for each membership -- 4.2. The model used in the simulation -- 4.3. Optimum control of the axial flow pump using a single pump speed -- 4.4. Optimum control of the axial flow pump using two pump speeds -- 5. Conclusion -- Acknowledgments -- References -- Chapter 2 Techniques in Visualization and Evaluation of the In Vivo Microcirculation Shigeru Ichioka -- 1. Introduction -- 2. Intravital Microscopic System -- 2.1. Microscope.
2.2. Suspension of the microscope -- 2.3. Illumination -- 2.4. Imaging and recording -- 3. Tissue Preparation -- 3.1. Anesthetics -- 3.2. Experimental models -- 3.2.1. Acute experimental models -- 3.2.2. Chronic experimental models -- 4. Microcirculatory Measurement -- 4.1. Vessel diameter -- 4.2. Tissue vascularity -- 4.3. Blood flow velocity -- 4.4. Leukocyte behavior -- 4.5. Oxygen measurement -- 4.6. Permeability measurement -- 5. Application of the Microcirculatory Experimental Models -- 5.1. Angiogenesis model -- 5.1.1. Angiogenesis model using mouse skinfold chamber -- 5.1.2. Angiogenesis model using hairless mouse ear -- 5.2. Ischemia-reperfusion -- References -- Chapter 3 Impedance Cardiography: Development of the Stroke Volume Equations and their Electrodynamic and Biophysical Foundations Donald Philip Bernstein and Hendrikus J. M. Lemmens -- 1. Introduction -- 2. Operational Implementation and Development of the Dual Compartment Parallel Conduction Model -- 3. Hemodynamic Biomechanical Analogs and Bioelectric Components of the Transthoracic Cardiogenic Impedance Pulse Variation Z(t) -- 3.1. The volumetric component: Zvol(t) -- 3.2. The blood velocity-induced "resistivity" component: Zv(t) -- 4. Development of the Kubicek and Sramek-Bernstein SV Equations -- 4.1. Nyboer equation: Foundation and rationale for the plethysmographic hypothesis in impedance cardiography -- 4.2. Kubicek equation -- 4.3. Assumptions implicit to the accuracy of Nyboer-Kubicek model -- 4.4. Sramek-Bernstein equation -- 4.5. Validity of the plethysmographic techniques -- 5. Theory and Rationale for a New Stroke Volume Equation -- 5.1. Analytical methods -- 5.2. Origin of dZ/dtmax -- 5.2.1. Resolution of origin by anatomic domain -- 5.2.2. Resolution of origin by differential time domain analysis.
5.2.3. Resolution of origin by comparative time domain analysis: time-to-peak (TTP) of dZ/dtmax -- 5.2.4. Hemodynamic and biomechanical origins of Z(t) and dZ/dtmax: Analysis by means of a dierentiable cylindrical model of ascending aortic blood flow -- 6. Rationale for Application of the Square Root Acceleration Step-down Transformation in Impedance Cardiography: Conversion of dZ/dtmax Normalized by its Base Impedance Z0 (dZ/dtmax/Z0) to Normalized Ohmic Mean Velocity Zv(t)max/Z0 -- 6.1. Peak aortic reduced average blood acceleration (PARABA): Hemodynamic analog of dZ/dtmax/Z0 -- 7. Stroke Volume Equation Implementing the Square Root Acceleration Step-down Transformation -- 7.1. The volume conductor -- 7.2. Index of transthoracic aberrant conduction ζ (zeta): Genesis of the three-compartment parallel conduction model -- 8. Proof of Hypothesis: The New Equation versus the Kubicek, Sramek, and Sramek-Bernstein Equations using Thermodilution as the Standard Reference Technique -- 9. Conclusions -- References -- Chapter 4 Indicator Dilution Techniques in Cardiovascular Quantification Massimo Mischi, Zaccaria Del Prete and Hendrikus H. M. Korsten -- 1. Introduction -- 2. The Cardiovascular System -- 3. Indicator Dilution Modeling -- 3.1. Overview of the indicator dilution models -- 3.2. The local density random walk model -- 3.3. The First Passage Time model -- 4. Cardiovascular Quantification by Indicator Dilution Principles -- 4.1. Cardiac output -- 4.2. Mean transit time and blood volumes -- 4.3. Ejection fraction -- 4.4. Myocardial perfusion -- 5. Indicator Dilution Techniques for Cardiovascular Quantification -- 5.1. Invasive techniques -- 5.1.1. Fick method -- 5.1.2. Thermodilution -- 5.1.3. Dye dilution -- 5.1.4. Lithium dilution -- 5.2. Indicator imaging techniques for cardiovascular quantification -- 5.2.1. Echocardiography.
5.2.2. X-ray angiography -- 5.2.3. Nuclear imaging -- 5.2.4. Magnetic resonance imaging -- 6. Conclusions and Outlook -- References -- Chapter 5 Analyzing Cardiac Biomechanics by Heart Sound Andreas Voss, R. Schroeder, A. Seeck and T. Huebner -- 1. Introduction -- 2. Background -- 2.1. The biomechanics of the healthy heart -- 2.2. Heart sounds and cardiac murmur -- 2.2.1. Heart sounds -- 2.2.1.1. The first heart sound -- 2.2.1.2. The second heart sound -- 2.2.2. Cardiac murmurs -- 2.3. The pathological heart -- 2.3.1. Cardiac arrhythmias -- 2.3.2. Coronary heart disease -- 2.3.3. Myocardial diseases -- 2.3.4. Heart defect -- 2.3.5. Heart valve diseases -- 2.3.5.1. Aortic valve stenosis -- 2.3.5.2. Aortic valve insufficiency -- 2.3.5.3. Mitral valve insufficiency -- 2.3.5.4. Tricuspid valve insufficiency -- 2.3.5.5. Pulmonary valve insufficiency -- 2.3.6. Biomechanical replacement systems -- 2.3.6.1. Replacement of the heart valves -- 2.3.6.2. Replacement systems for cardiac arrhythmia -- 2.3.6.3. Cardiac assist device and artificial heart -- 3. Methods of Analyzing Cardiac Biomechanics -- 3.1. Computer tomography (CT) and magnetic resonance tomography (MRT) -- 3.2. Ultrasound - echocardiography -- 3.3. Heart sound - auscultation and phonocardiography -- 4. Heart Sound Evaluation for Analyzing Cardiac Biomechanics -- 4.1. Stethoscopes and heart sound sensors -- 4.1.1. State of the art -- 4.1.2. Design of a new electronic stethoscope -- 4.2. Heart sound analysis for detection of biomechanical disorders of the heart valves -- 4.2.1. State of the art -- 4.2.2. Heart sound analysis based on feature extraction -- 4.2.2.1. Signal recording and preliminary processing -- 4.2.2.2. Parameter extraction -- 4.2.2.3. Using the methods for analysis of heart sounds in patients with heart valve disease -- 4.2.2.4. Discussion.
4.3. Heart sound analysis for risk stratification in patients with heart failure -- 5. Discussion -- References -- Chapter 6 Methods in the Analysis of the Effects of Gravity and Wall Properties in Blood Flow Through Vascular Systems S. J. Payne and S. Uzel -- 1. Introduction -- 2. Theory -- 2.1. Fluid dynamic equations -- 2.1.1. One-dimensional flow -- 2.1.2. Two-dimensional flow -- 2.2. Vessel wall equations -- 2.2.1. Two-dimensional deformation -- 2.2.2. One-dimensional deformation -- 2.2.3. Existing pressure-area relationships -- 2.3. Coupled form of equations -- 3. Model Predictions -- 3.1. Steady state behavior -- 3.2. Wave behavior -- 4. Discussion -- 5. Conclusions -- Acknowledgments -- References -- Chapter 7 Numerical and Experimental Techniques for the Study of Biomechanics in the Arterial System Thomas P. O'Brien, Michael T. Walsh, Liam Morris, Pierce A. Grace, Eamon G. Kavanagh and Tim M. McGloughlin -- 1. Introduction -- 2. Medical Imaging Techniques -- 2.1. Magnetic resonance imaging -- 2.1.1. Operating principles -- 2.1.2. Application -- 2.2. Computerized tomography -- 2.2.1. Operating principles -- 2.2.2. Application -- 2.3. Ultrasound -- 2.3.1. Operating principle -- 2.3.2. Application -- 2.4. Angiography -- 3. Computer Aided Design and Manufacture -- 3.1. Digital image processing -- 3.1.1. Process application -- 3.1.2. Velocity profile processing -- 3.1.3. Vector transformations -- 3.2. Rapid prototyping -- 3.2.1. Application -- 3.3. Casting -- 3.3.1. Application -- 4. Numerical Investigations -- 4.1. Computational fluid dynamics -- 4.1.1. Application -- 4.2. Finite element analysis -- 4.2.1. Application -- 5. Experimental Investigations -- 5.1. Physiological flow modeling -- 5.2. Laser Doppler anemometry -- 5.3. Particle image velocimetry -- 5.4. Video extensometry -- 5.5. Pressure and flow-rate measurement -- 5.6. Photoelasticity.
6. Medical Device Research and Development.
Abstract:
Because of rapid developments in computer technology and computational techniques, advances in a wide spectrum of technologies, coupled with cross-disciplinary pursuits between technology and its application to human body processes, the field of biomechanics continues to evolve. Many areas of significant progress include dynamics of musculoskeletal systems, mechanics of hard and soft tissues, mechanics of bone remodeling, mechanics of blood and air flow, flow-prosthesis interfaces, mechanics of impact, dynamics of man-machine interaction, and more. Thus, the great breadth and significance of the field in the international scene require a well integrated set of volumes to provide a complete coverage of the exciting subject of biomechanical systems technology. World-renowned contributors tackle the latest technologies in an in-depth and readable manner. -->. Sample Chapter(s). Chapter 1: A Simulation Study of Hemodynamic Benefits and Optimal Control of Axial Flow Pump-Based Left Ventricular Assist. Contents: Techniques in Visualization and Evaluation of the In Vivo Microcirculation (S Ichioka); Analyzing Cardiac Biomechanics by Heart Sound (A Voss et al.); Numerical and Experimental Techniques for the Study of Biomechanics in the Arterial System (T P O'Brien et al.); and many other papers. Readership: Academics, researchers and postgraduate students in anatomy, cardiology, orthopaedic, biomechanics and surgery.
Local Note:
Electronic reproduction. Ann Arbor, Michigan : ProQuest Ebook Central, 2017. Available via World Wide Web. Access may be limited to ProQuest Ebook Central affiliated libraries.
Genre:
Electronic Access:
Click to View