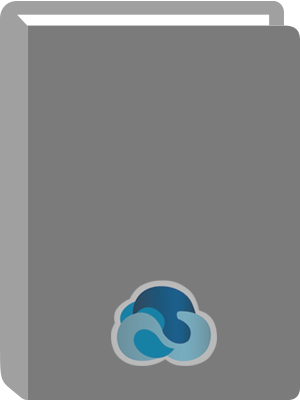
Advanced Composite Materials for Automotive Applications : Structural Integrity and Crashworthiness.
Title:
Advanced Composite Materials for Automotive Applications : Structural Integrity and Crashworthiness.
Author:
Elmarakbi, Ahmed.
ISBN:
9781118535271
Personal Author:
Edition:
1st ed.
Physical Description:
1 online resource (472 pages)
Series:
Automotive Series
Contents:
ADVANCED COMPOSITE MATERIALS FOR AUTOMOTIVE APPLICATIONS -- Contents -- About the Editor -- List of Contributors -- Series Preface -- Preface -- Part One FUNDAMENTAL BACKGROUND -- 1 Overview of Composite Materials and their Automotive Applications -- 1.1 Introduction -- 1.2 Polymer Composite Materials -- 1.2.1 Non-Structural Composites -- 1.2.2 Semi-Structural Composites -- 1.2.3 Structural Composites -- 1.2.4 Laminated Composites -- 1.2.5 Textile Composites -- 1.2.6 Hybrid Composites -- 1.3 Application of Composite Materials in the Automotive Industry -- 1.3.1 Crashworthiness -- 1.3.2 Composite Driveshaft and Spring -- 1.3.3 Other Applications -- 1.4 Green Composites for Automotive Applications -- 1.5 Modelling the Mechanical Behaviour of Composite Materials -- 1.5.1 Modelling the Elastic Properties of Unidirectional Composites -- 1.5.2 Modelling of Laminated and Textile Composites -- 1.5.2.1 Analytical Modelling -- 1.5.2.2 Numerical FE Modelling -- 1.6 Discussion -- 1.7 Conclusion -- References -- 2 High-Volume Thermoplastic Composite Technology for Automotive Structures -- 2.1 Introduction - Opportunities for Thermoplastic Composites -- 2.2 Recent Developments in Automotive TPCs -- 2.3 Case Study: Rapid Stamp-Formed Thermoplastic Composites -- 2.3.1 Materials Selection: Exploring the Potential of Aligned Fibre TPCs -- 2.3.2 Demonstrator Beam Component -- 2.3.3 TPC Process Development -- 2.3.4 Beam Manufacture -- 2.3.5 Demonstrator Beam Structural Performance -- 2.3.6 Environmental Impact Assessment -- 2.3.7 Economic Analysis -- 2.4 Conclusion -- Acknowledgements -- References -- 3 Development of Low-Cost Carbon Fibre for Automotive Applications -- 3.1 Introduction -- 3.2 Research Drivers: Energy Efficiency -- 3.3 Lightweight Automotive Materials -- 3.4 Barriers to Carbon Fibre Adoption in the Automotive Industry.
3.5 Global Production and the Market for Carbon Fibre -- 3.6 Low-Cost Carbon Fibre Programme -- 3.6.1 Project Aims -- 3.6.2 Precursor Materials -- 3.6.2.1 Commodity PAN-Based Precursors -- 3.6.2.2 Lignin-Based Precursors -- 3.6.2.3 Polyolefin-Based Precursors -- 3.6.3 Advanced Processing Techniques -- 3.6.3.1 Microwave Assisted Plasma Processing -- 3.6.3.2 Advanced Stabilisation/Crosslinking -- 3.6.3.3 Plasma Oxidation -- 3.6.3.4 Advanced Surface Treatment and Sizing -- 3.6.4 Integration: Low-Cost Carbon Fibre Pilot Line -- 3.7 International Cooperation -- Acknowledgements -- References -- Part Two IMPACT AND CRASH ANALYSIS -- 4 Mechanical Properties of Advanced Pore Morphology Foam Composites -- 4.1 Introduction -- 4.2 Cellular Materials -- 4.2.1 Mechanical Behaviour of Cellular Materials -- 4.2.2 Energy Absorption Capabilities of Cellular Materials -- 4.2.3 Influence of Pore Fillers -- 4.2.4 Strain Rate Sensitivity of Cellular Materials -- 4.3 Advanced Pore Morphology Foam -- 4.4 Mechanical Properties of Single APM Foam Elements -- 4.5 Behaviour of Composite APM Foam -- 4.5.1 Compressive Loading of Confined APM Foam Elements without Bonding -- 4.5.2 Partially Bonded APM Foam Elements -- 4.5.3 Fully Bonded APM Foam Elements - Syntactic Structure -- 4.6 Conclusion -- Acknowledgements -- References -- 5 Automotive Composite Structures for Crashworthiness -- 5.1 Introduction -- 5.2 Traffic Safety -- 5.3 Alternative Vehicles -- 5.4 Selective Overview of Worldwide Crash Tests -- 5.5 Structural Crash Management -- 5.5.1 Front Crash -- 5.5.2 Side Crash -- 5.6 Composite Materials for Crash Applications -- 5.6.1 Performance Metrics for Energy Absorbing Structures -- 5.6.2 Energy Absorbing Deformation Mechanisms in Composite Profiles -- 5.7 Energy Absorption of Composite Profiles -- 5.7.1 Fibre Material -- 5.7.2 Matrix Material -- 5.7.3 Fibre Volume Fraction.
5.7.4 Fibre Architecture -- 5.7.5 Trigger -- 5.7.6 Geometry -- 5.7.7 Test Speed -- 5.7.8 Test Direction -- 5.8 Conclusion -- Acknowledgements -- References -- 6 Crashworthiness Analysis of Composite and Thermoplastic Foam Structure for Automotive Bumper Subsystem -- 6.1 Introduction -- 6.2 Materials for Automotive Applications -- 6.3 Composite and Thermoplastic Materials -- 6.4 Numerical Modelling of Fiat 500 Frontal Transverse Beam -- 6.5 Standards for Low-Speed Frontal Impact -- 6.6 Bumper Beam Thickness Determination -- 6.7 Results and Discussion -- 6.8 Conclusion -- References -- 7 Hybrid Structures Consisting of Sheet Metal and Fibre Reinforced Plastics for Structural Automotive Applications -- 7.1 Introduction and Motivation -- 7.2 Conventional Method for the Development of Composite Structures -- 7.3 Approaches to Automotive Lightweight Construction -- 7.4 Requirements for Automotive Structures -- 7.4.1 Mechanical Requirements -- 7.4.2 Load Adapted Design -- 7.4.3 Derivation of Reference Structures -- 7.5 Simulation -- 7.6 Manufacturing -- 7.6.1 Overview -- 7.6.2 Prepreg Press Technology: Basic Investigations and Process Parameters -- 7.6.3 Prepreg Press Technology: Bonding of Composite Material and Sheet Metal -- 7.7 Testing -- 7.7.1 Quasi-Static Tests -- 7.7.2 Crash Tests -- 7.8 New Methodology for the Product Engineering of Hybrid Lightweight Structures -- 7.9 Conclusion -- References -- 8 Nonlinear Strain Rate Dependent Micro-Mechanical Composite Material Model for Crashworthiness Simulation -- 8.1 Introduction -- 8.2 Micro-Mechanical Formulation -- 8.2.1 Equations for Micro-Mechanical Model -- 8.2.1.1 Constitutive Equations for Composite Materials -- 8.2.1.2 Micro-Mechanics Constitutive Model -- 8.2.1.3 Constitutive Matrices and Stress Update for the Micro-Model -- 8.2.2 Failure Analysis -- 8.2.3 Finite Element Implementation.
8.2.3.1 Equations in Incremental Form -- 8.2.3.2 Localisation and Modification -- 8.2.4 Verification Examples -- 8.3 Strain Rate Dependent Effects -- 8.3.1 Strain Rate Effect Introduction and Review -- 8.3.1.1 Strain Rate Effect on Polymer Resin -- 8.3.1.2 State Variable Modelling Overview -- 8.3.2 One-Dimensional Equation and Material Constant Determination -- 8.3.2.1 One-Dimensional Constitutive Equation -- 8.3.2.2 Material Constant Determination -- 8.3.3 Three-Dimensional Constitutive Equations -- 8.3.3.1 Original Flow Equation -- 8.3.3.2 Modified Equations with Shear Correction Factor -- 8.3.3.3 Three-Dimensional Extension of Internal Stress Evolution Law -- 8.3.4 Finite Element Implementation -- 8.3.4.1 Shell Element Simulation -- 8.3.4.2 Solid Element Simulation -- 8.4 Numerical Results -- 8.5 Conclusion -- References -- 9 Design Solutions to Improve CFRP Crash-Box Impact Efficiency for Racing Applications -- 9.1 Introduction -- 9.2 Composite Structures for Crashworthy Applications -- 9.3 Geometrical and Material Characterisation of the Impact Attenuator -- 9.4 Experimental Test -- 9.5 Finite Element Analysis and LS-DYNA -- 9.6 Comparison between Numerical and Experimental Analysis -- 9.7 Investigation of the Optimal Solution -- 9.8 Conclusion -- References -- Part Three DAMAGE AND FAILURE -- 10 Fracture and Failure Mechanisms for Different Loading Modes in Unidirectional Carbon Fibre/Epoxy Composites -- 10.1 Introduction -- 10.2 Delamination Failure -- 10.3 Objectives -- 10.4 Experimental Programme -- 10.4.1 Materials and Laminate Manufacturing -- 10.4.2 Testing Methods -- 10.4.2.1 Mode I Test Method -- 10.4.2.2 Mixed Mode I/II: ADCB Test -- 10.4.2.3 Mixed Mode I/II: MMB Test -- 10.4.2.4 Mode II Test Method -- 10.5 Numerical Simulations -- 10.5.1 Virtual Crack Closure Technique -- 10.5.2 Two-Step Extension Method -- 10.5.3 Cohesive Zone Model.
10.6 Fractography -- 10.7 Results and Discussion -- 10.7.1 Experimental Results -- 10.7.2 Numerical Results -- 10.7.3 Fractographic Analysis -- 10.7.4 Stress State at the Crack Front -- 10.8 Conclusion -- References -- 11 Numerical Simulation of Damages in FRP Laminated Structures under Transverse Quasi-Static or Low-Velocity Impact Loads -- 11.1 Introduction -- 11.2 Theory -- 11.2.1 Theory of Finite Element Method -- 11.2.2 Damage Models -- 11.2.2.1 In-Plane Damage -- 11.2.2.2 Theory of Traditional Cohesive Element for Modelling Delamination -- 11.3 Techniques for Overcoming Numerical Instability in Simulation of Delamination Propagation -- 11.3.1 Artificial Damping Technique -- 11.3.2 Move-Limit Technique Enforced on Cohesive Zone -- 11.3.3 Adaptive Cohesive Model -- 11.3.3.1 Rate-Independent Adaptive Cohesive Model -- 11.3.3.2 Rate-Dependent Adaptive Cohesive Model -- 11.4 Numerical Examples -- 11.4.1 DCB Problem -- 11.4.1.1 Standard Numerical Simulations -- 11.4.1.2 Artificial Damping Technique -- 11.4.1.3 Move-Limit Technique -- 11.4.1.4 Rate-Independent ACM -- 11.4.1.5 Rate-Dependent ACM -- 11.4.2 Low-Velocity Impact Problem -- 11.5 Conclusion -- References -- 12 Building Delamination Fracture Envelope under Mode I/Mode II Loading for FRP Composite Materials -- 12.1 Introduction -- 12.2 Experimental Studies -- 12.3 Mode I Delamination Testing: Double Cantilever Bending Test Analysis and Results -- 12.4 Mode II Delamination Testing: End Notched Flexure Test Analysis and Results -- 12.5 Mixed Mode I/II Delamination Testing: Mixed-Mode Bending Test Analysis and Results -- 12.6 Fracture Failure Envelope -- 12.7 Conclusion -- Nomenclature -- References -- Part Four CASE STUDIES AND DESIGNS -- 13 Metal Matrix Composites for Automotive Applications -- 13.1 Automotive Technologies -- 13.1.1 Current Landscape -- 13.1.2 Alternative Technologies.
13.1.2.1 Hybrid Vehicles.
Abstract:
The automotive industry faces many challenges, including increased global competition, the need for higher-performance vehicles, a reduction in costs and tighter environmental and safety requirements. The materials used in automotive engineering play key roles in overcoming these issues: ultimately lighter materials mean lighter vehicles and lower emissions. Composites are being used increasingly in the automotive industry due to their strength, quality and light weight. Advanced Composite Materials for Automotive Applications: Structural Integrity and Crashworthiness provides a comprehensive explanation of how advanced composite materials, including FRPs, reinforced thermoplastics, carbon-based composites and many others, are designed, processed and utilized in vehicles. It includes technical explanations of composite materials in vehicle design and analysis and covers all phases of composite design, modelling, testing and failure analysis. It also sheds light on the performance of existing materials including carbon composites and future developments in automotive material technology which work towards reducing the weight of the vehicle structure. Key features: Chapters written by world-renowned authors and experts in their own fields Includes detailed case studies and examples covering all aspects of composite materials and their application in the automotive industries Unique topic integration between the impact, crash, failure, damage, analysis and modelling of composites Presents the state of the art in composite materials and their application in the automotive industry Integrates theory and practice in the fields of composite materials and automotive engineering Considers energy efficiency and environmental implications Advanced Composite Materials for Automotive Applications: Structural Integrity and Crashworthiness is a comprehensive
reference for those working with composite materials in both academia and industry, and is also a useful source of information for those considering using composites in automotive applications in the future.
Local Note:
Electronic reproduction. Ann Arbor, Michigan : ProQuest Ebook Central, 2017. Available via World Wide Web. Access may be limited to ProQuest Ebook Central affiliated libraries.
Genre:
Electronic Access:
Click to View