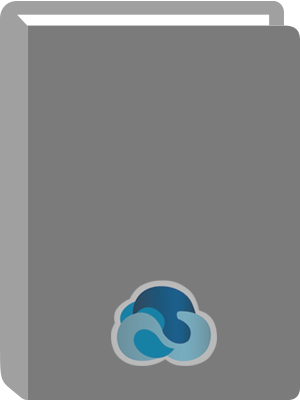
Fusion Protein Technologies for Biopharmaceuticals : Applications and Challenges.
Title:
Fusion Protein Technologies for Biopharmaceuticals : Applications and Challenges.
Author:
Schmidt, Stefan R.
ISBN:
9781118354582
Personal Author:
Edition:
1st ed.
Physical Description:
1 online resource (1067 pages)
Contents:
Fusion Protein Technologies for Biopharmaceuticals: Applications and Challenges -- Contents -- Preface -- Contributors -- PART I: INTRODUCTION -- 1 Fusion Proteins: Applications and Challenges -- 1.1 History -- 1.2 Definitions and Categories -- 1.3 Patenting -- 1.4 Design and Engineering -- 1.4.1 Orientation of Fusion Proteins -- 1.4.2 Linker Engineering -- 1.4.3 Oligomerization of Fusion Proteins -- 1.4.4 Immunogenicity -- 1.4.5 Mutagenesis for Molecule Optimization -- 1.5 Manufacturing -- 1.5.1 Upstream Process -- 1.5.2 Downstream Process -- 1.5.3 Formulation -- 1.5.4 Process Economies -- 1.5.5 Glycosylation -- 1.6 Regulatory Challenges -- 1.7 Competition and Market -- 1.8 Conclusion and Future Perspective -- References -- 2 Analyzing and Forecasting the Fusion Protein Market and Pipeline -- 2.1 Introduction -- 2.2 Market Sales Dynamics of the FP Market -- 2.2.1 Enbrel Founded the FP Market Following Its Launch in 1998 -- 2.2.2 We Forecast the FP Market Will Grow to 10.1 Billion by 2016 -- 2.2.3 Growth Drivers and Restrictors that Impact the FP Market -- 2.3 Individual Drug Sales Analysis -- 2.3.1 Enbrel -- 2.3.2 Orencia -- 2.3.3 Nplate -- 2.3.4 Dulaglutide and Albiglutide -- 2.3.5 VEGFtrap-Eye -- 2.4 Pipeline Database Analysis -- 2.4.1 Breakdown by Stage of Development: Half of the FP Pipeline is in Phase II -- 2.4.2 Therapeutic Focus of FPs: Oncology and Autoimmune Dominate -- 2.4.3 FPs Developers and Partners: Many Big Pharma/Big Biotech Companies Have at Least One FP in Phase II Development or Above -- 2.4.4 FP Development: Less Attractive for Earlier-Stage Drug Developers, but More Attractive for Big Pharma Licensees -- 2.4.5 FPs Deal Analysis: We Believe FPs are an Attractive Target for Multinational Drug Companies -- Disclaimer -- Acknowledgment -- References.
3 Structural Aspects of Fusion Proteins Determining the Level of Commercial Success -- 3.1 Classification of FPs -- 3.1.1 Pharmacokinetic, Targeting, and Activity FP Components -- 3.1.2 FPs Classified by the Function of Each Component -- 3.1.3 Half-Life Extension Represents a Dominant Theme (1c-7c) -- 3.1.3.1 Fc Fusion is the Half-Life Extension Standard -- 3.1.3.2 Albumin Fusion is the Most Advanced non-FcFP Half-Life Approach -- 3.1.3.3 Nonfusion Alternatives -- 3.1.4 Decoy Receptors are the Largest FP Group and Well Validated (1c) -- 3.1.5 Extended Half-Life Ligands (4c) -- 3.1.6 FPs Offer a Wide Range of Cell Death Mechanisms (3a-7b) -- 3.1.6.1 Targeted Toxins (3a and 3b) -- 3.1.6.2 Targeted Ligands (4a and 4b) -- 3.1.6.3 Antigen-Mediated Cell Death (5a and 5b) -- 3.1.7 Antibody Fragment Fusions (6b and 7b) -- 3.1.7.1 Bispecific Abs: Ab-Binding Domain Fused to Ab-Binding Domain (6b) -- 3.1.7.2 Miniaturized Abs: Ab-Binding Domain Fused to Fc Effector (7b) -- 3.1.7.3 Other Ab Fragment Structures -- 3.1.8 Ab- and non-Ab-Targeting Decisions -- 3.1.9 Targeted Enzymes (2a) -- 3.2 Factors for Commercial Success -- 3.2.1 Administration -- 3.2.2 Efficacy Profile -- 3.2.3 Safety Profile -- 3.2.4 Cost Factors -- 3.2.4.1 Manufacturing and Cost of Goods Sold -- 3.2.4.2 Intellectual Property: Freedom to Operate and Protection from Copies -- References -- 4 Fusion Protein Linkers: Effects on Production, Bioactivity, and Pharmacokinetics -- 4.1 Introduction -- 4.2 Overview of General Properties of Linkers Derived From Naturally Occurring Multidomain Proteins -- 4.2.1 Length of Linker Sequence -- 4.2.2 Preference of Amino Acid Residues in Linker Sequence -- 4.2.3 Hydrophobicity of Linkers -- 4.2.4 Secondary Structures of Linkers -- 4.3 Empirical Linkers in Recombinant Fusion Proteins -- 4.3.1 Flexible Linkers -- 4.3.1.1 Flexible Glycine and Serine Linkers.
4.3.1.2 Other Flexible Linkers -- 4.3.2 Rigid Linkers -- 4.3.2.1 Alpha Helix-Forming Linker (EAAAK)n -- 4.3.2.2 Rigid (XP)n Linkers -- 4.3.3 In Vivo Cleavable Linkers -- 4.3.3.1 In Vivo Cleavable Disulfide Linker -- 4.3.3.2 In Vivo Cleavable Protease-Sensitive Linkers -- 4.4 Functionality of Linkers in Fusion Proteins -- 4.4.1 Linkers Can Improve Biological Activity of Fusion Proteins -- 4.4.2 Linkers Can Increase Expression Yield of Fusion Proteins -- 4.4.3 Linkers Can Affect the PK of Fusion Proteins -- 4.5 Conclusions and Future Perspective -- References -- 5 Immunogenicity of Therapeutic Fusion Proteins: Contributory Factors and Clinical Experience -- 5.1 Introduction -- 5.2 Basis of Therapeutic Protein Immunogenicity -- 5.2.1 Relevance to Fusion Proteins -- 5.3 Tools for Immunogenicity Screening -- 5.3.1 In Silico Analysis Tools -- 5.3.2 In Vitro Analysis Tools -- 5.4 Approaches for Risk Assessment and Minimization -- 5.4.1 Importance to Product Development -- 5.4.2 De-immunization by Epitope Modification -- 5.4.3 Tolerance Induction -- 5.4.4 Regulatory T-Cell Epitopes in IgG Framework Regions: Tregitopes -- 5.5 Case Study and Clinical Experience -- 5.5.1 Observed Versus Predicted Immunogenicity of 21 Antibodies in Clinical Use -- 5.5.2 Correlation of Clinical Immunogenicity of Fusion Proteins with In Silico Risk Estimates -- 5.6 Preclinical and Clinical Immunogenicity Assessment Strategy -- 5.6.1 Strategy and Recommendation -- 5.7 Conclusions -- Acknowledgment -- References -- PART II THE TRIPLE T PARADIGM: TIME, TOXIN, TARGETING -- IIA TIME: FUSION PROTEIN STRATEGIES FOR HALF-LIFE EXTENSION -- 6 Fusion Proteins for Half-Life Extension -- 6.1 Introduction -- 6.2 Half-Life Extension Through Size and Recycling -- 6.2.1 Direct Genetic Fusions -- 6.2.1.1 Albumin Fusions -- 6.2.1.2 Fc Fusions -- 6.2.1.3 Transferrin Fusions.
6.2.2 Half-Life Extension Through Attachment to Large Proteins -- 6.3 Half-Life Extension Through Increase of Hydrodynamic Radius -- 6.3.1 Repetitive Peptide Fusions -- 6.3.2 Glycosylated Peptides -- 6.4 Aggregate Forming Peptide Fusions -- 6.5 Other Concepts -- 6.6 Conclusions and Future Perspective -- References -- 7 Monomeric Fc-Fusion Proteins -- 7.1 Introduction -- 7.2 FcRn and Monomeric Fc-Fusion Proteins -- 7.2.1 FcRn Expression and Function -- 7.2.2 FcRn for the Delivery of Fc-Fusion Proteins -- 7.3 Typical Applications -- 7.3.1 Interferon Beta-Fc Monomer -- 7.3.2 Interferon α-Fc Monomer -- 7.3.3 Factor VIII-Fc Monomer -- 7.3.4 Factor IX-Fc Monomer -- 7.4 Alternative Applications -- 7.4.1 Follicle Stimulating Hormone-Fc-Fusion Proteins -- 7.4.2 Cytokine-Fc-Fusion Proteins -- 7.5 Expression and Purification of Monomeric Fc-Fusion Proteins -- 7.6 Conclusions and Future Perspectives -- References -- 8 Peptide-Fc Fusion Therapeutics: Applications and Challenges -- 8.1 Introduction -- 8.2 Peptide Drugs -- 8.2.1 The Limitations of Native Endogenous Peptides in Drug Development -- 8.2.2 Chemical Modification of Peptides to Reduce Proteolytic Degradation -- 8.2.3 Constraining Peptides to be More Resistant to Protease Cleavage -- 8.2.4 Peptide Therapeutics Play an Important Role in Drug Development -- 8.2.5 Most Peptide Drugs Act as Receptor Agonists -- 8.3 Technologies Used for Reducing In Vivo Clearance of Therapeutic Peptides -- 8.3.1 PEGylation -- 8.3.2 Human Serum Albumin Fusions -- 8.3.3 Other Approaches -- 8.4 Fc-Fusion Proteins in Drug Development -- 8.4.1 The Structure of Fc-Fusion Proteins -- 8.4.2 Fc-Fusion Therapeutics -- 8.4.2.1 Enbrel® -- 8.4.2.2 Amevive® -- 8.4.2.3 Orencia® -- 8.4.2.4 Arcalyst® (Also Known as IL-1 Trap or Rilonacept) -- 8.4.2.5 Other Fc-Fusion Protein Therapeutics -- 8.5 Peptide-Fc-Fusion Therapeutics -- 8.5.1 Peptibody.
8.5.1.1 NPlate® (Romiplostim, AMG531) -- 8.5.1.2 AMG 386 (2xCon4(C)) -- 8.5.1.3 A-623 (AMG 623) -- 8.5.1.4 Dulaglutide (LY2189265) -- 8.5.2 MimetibodyTM Technology -- 8.5.2.1 CNTO 528/CNTO 530 -- 8.5.2.2 CNTO736 -- 8.6 Considerations and Challenges for Engineering Peptide-Fc-Fusion Therapeutics -- 8.6.1 Improving Activity -- 8.6.2 Improving Stability -- 8.6.3 Improving Pharmacokinetic Profile -- 8.6.4 Improving Solubility and Reducing Aggregation -- 8.6.5 Reducing Heterogeneity -- 8.6.6 Isotype Selection -- 8.6.7 Tailoring Fc-Effector Functions to Fit Individual Needs -- 8.6.8 Reducing Immunogenicity -- 8.7 Conclusions -- Acknowledgment -- References -- 9 Receptor-Fc and Ligand Traps as High-Affinity Biological Blockers: Development and Clinical Applications -- 9.1 Introduction -- 9.2 Etanercept as a Prototypical Receptor-Fc-Based Cytokine Blocker -- 9.3 Heteromeric Traps for Ligands Utilizing Multicomponent Receptor Systems with Shared Subunits -- 9.3.1 Proof-of-Concept for High-Affinity Traps for Ligands Utilizing Multicomponent Receptor Systems -- 9.3.2 Turning Heterodimeric Soluble Receptor-Based Ligand Traps into Therapeutics -- 9.4 Development and Clinical Application of an Interleukin 1 Trap: Rilonacept -- 9.5 Development and Clinical Application of a VEGF Trap -- 9.6 "To Trap Or Not To Trap?" Advantages and Disadvantages of Receptor-Fc Fusions and Traps Versus Antibodies -- 9.6.1 Multiple Ligand Binding -- 9.6.2 Signaling of Receptor-Fc Fusions -- 9.6.3 Immunogenicity -- 9.7 Conclusion -- Acknowledgment -- References -- 10 Recombinant Albumin Fusion Proteins -- 10.1 Concept -- 10.2 Technological Aspects -- 10.3 Typical Applications and Indications -- 10.3.1 Albumin Fusion Proteins in Late-Stage Development -- 10.3.1.1 Interferon-α -- 10.3.1.2 Glucagon-Like Peptide-1 -- 10.3.1.3 Granulocyte Colony-Stimulating Factor.
10.3.2 Albumin Fusion Proteins in Early-Stage Development.
Abstract:
The state of the art in biopharmaceutical FUSION PROTEIN DESIGN Fusion proteins belong to the most lucrative biotech drugs-with Enbrel® being one of the best-selling biologics worldwide. Enbrel® represents a milestone of modern therapies just as Humulin®, the first therapeutic recombinant protein for human use, approved by the FDA in 1982 and Orthoclone® the first monoclonal antibody reaching the market in 1986. These first generation molecules were soon followed by a plethora of recombinant copies of natural human proteins, and in 1998, the first de novo designed fusion protein was launched. Fusion Protein Technologies for Biopharmaceuticals examines the state of the art in developing fusion proteins for biopharmaceuticals, shedding light on the immense potential inherent in fusion protein design and functionality. A wide pantheon of international scientists and researchers deliver a comprehensive and complete overview of therapeutic fusion proteins, combining the success stories of marketed drugs with the dynamic preclinical and clinical research into novel drugs designed for as yet unmet medical needs. The book covers the major types of fusion proteins-receptor-traps, immunotoxins, Fc-fusions and peptibodies-while also detailing the approaches for developing, delivering, and improving the stability of fusion proteins. The main body of the book contains three large sections that address issues key to this specialty: strategies for extending the plasma half life, the design of toxic proteins, and utilizing fusion proteins for ultra specific targeting. The book concludes with novel concepts in this field, including examples of highly relevant multifunctional antibodies. Detailing the innovative science, commercial realities, and brilliant potential of fusion protein therapeutics, Fusion Protein Technologies for Biopharmaceuticals is a must for
pharmaceutical scientists, biochemists, medicinal chemists, molecular biologists, pharmacologists, and genetic engineers interested in determining the shape of innovation in the world of biopharmaceuticals.
Local Note:
Electronic reproduction. Ann Arbor, Michigan : ProQuest Ebook Central, 2017. Available via World Wide Web. Access may be limited to ProQuest Ebook Central affiliated libraries.
Genre:
Electronic Access:
Click to View