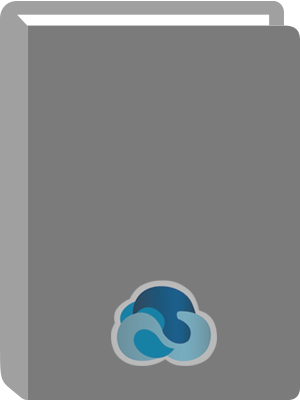
Electrocatalysis : Theoretical Foundations and Model Experiments, Volume 14.
Title:
Electrocatalysis : Theoretical Foundations and Model Experiments, Volume 14.
Author:
Alkire, Richard C.
ISBN:
9783527680467
Personal Author:
Edition:
1st ed.
Physical Description:
1 online resource (321 pages)
Series:
Advances in Electrochemical Sciences and Engineering ; v.28
Advances in Electrochemical Sciences and Engineering
Contents:
Electrocatalysis: Theoretical Foundations and Model Experiments -- Contents -- Preface -- List of Contributors -- 1 Multiscale Modeling of Electrochemical Systems -- 1.1 Introduction -- 1.2 Introduction to Multiscale Modeling -- 1.3 Electronic Structure Modeling -- 1.3.1 Modern Electronic Structure Theory -- 1.3.1.1 Quantum Mechanical Foundations -- 1.3.1.2 Born-Oppenheimer Approximation -- 1.3.1.3 Single-Electron Hamiltonians -- 1.3.1.4 Basis Sets -- 1.3.1.5 Enforcing the Pauli Principle -- 1.3.1.6 Electron Correlation Methods -- 1.3.1.7 Density Functional Theory -- 1.3.2 Applications of Electronic Structure to Geometric Properties -- 1.3.2.1 Geometry Optimization -- 1.3.2.2 Transition State Searches -- 1.3.3 Corrections to Potential Energy Surfaces and Reaction Pathways -- 1.3.3.1 Energy and Entropy Corrections -- 1.3.3.2 Thermodynamic State Functions -- 1.3.3.3 Reaction Energies and Rates -- 1.3.4 Electronic Structure Models in Electrochemistry -- 1.3.4.1 Modeling the Electrode Surface: Cluster versus Slab -- 1.3.4.2 Modeling the Solvent: Explicit versus Implicit -- 1.3.4.3 Modeling the Electrode Potential -- 1.3.5 Summary -- 1.4 Molecular Simulations -- 1.4.1 Energy Terms and Force Field Parameters -- 1.4.1.1 Covalent Bond Interactions -- 1.4.1.2 Non-Covalent Interactions -- 1.4.2 Parametrization and Validation -- 1.4.3 Atomistic Simulations -- 1.4.3.1 Monte Carlo Methods -- 1.4.3.2 Molecular Dynamics -- 1.4.3.3 QM/MM -- 1.4.4 Sampling and Analysis -- 1.4.5 Applications of Molecular Modeling in Electrochemistry -- 1.4.6 Summary -- 1.5 Reaction Modeling -- 1.5.1 Introduction -- 1.5.2 Chemical Kinetics -- 1.5.3 Kinetic Monte Carlo -- 1.5.3.1 System States and the Lattice Approximation -- 1.5.3.2 Reaction Rates -- 1.5.3.3 Reaction Dynamics -- 1.5.3.4 Applications of kMC in Electrochemistry -- 1.5.4 Summary.
1.6 The Oxygen Reduction Reaction on Pt(111) -- 1.6.1 Introduction to the Oxygen Reduction Reaction -- 1.6.2 Preliminary Considerations -- 1.6.3 DFT Calculations -- 1.6.4 Method Validation -- 1.6.5 Reaction Energies -- 1.6.6 Solvation Effects -- 1.6.7 Free Energy Contributions -- 1.6.8 Influence of an Electrode Potential -- 1.6.9 Modeling the Kinetic Rates -- 1.6.10 Summary -- 1.7 Formic Acid Oxidation on Pt(111) -- 1.7.1 Introduction to Formic Acid Oxidation -- 1.7.2 Density Functional Theory Calculations -- 1.7.3 Gas Phase Reactions -- 1.7.4 Explicit Solvation Model -- 1.7.5 Eley-Rideal Mechanisms and the Electrode Potential -- 1.7.6 Kinetic Rate Model of Formic Acid Oxidation -- 1.7.7 Summary -- 1.8 Concluding Remarks -- References -- 2 Statistical Mechanics and Kinetic Modeling of Electrochemical Reactions on Single-Crystal Electrodes Using the Lattice-Gas Approximation -- 2.1 Introduction -- 2.2 Lattice-Gas Modeling of Electrochemical Surface Reactions -- 2.3 Statistical Mechanics and Approximations -- 2.3.1 Static System -- 2.3.2 Dynamical System -- 2.4 Monte Carlo Simulations -- 2.5 Applications to Electrosorption, Electrodeposition and Electrocatalysis -- 2.5.1 Electrosorption and Electrodeposition -- 2.5.2 Electrocatalysis -- 2.6 Conclusions -- References -- 3 Single Molecular Electrochemistry within an STM -- 3.1 Introduction -- 3.2 Experimental Methods for Single Molecule Electrical Measurements in Electrochemical Environments -- 3.3 Electron Transfer Mechanisms -- 3.3.1 Tunneling -- 3.3.2 Resonant Tunneling -- 3.3.3 Hopping Models -- 3.4 Single Molecule Electrochemical Studies with an STM -- 3.4.1 Adsorbed Iron Complexes -- 3.4.2 Viologens -- 3.4.3 Osmium and Cobalt Metal Complexes -- 3.4.4 PyrroloTTF (pTTF) -- 3.4.5 Perylene Tetracarboxylic Diimides -- 3.4.6 Oligo(phenylene ethynylene) Derivates -- 3.5 Conclusions and Outlook.
References -- 4 From Microbial Bioelectrocatalysis to Microbial Bioelectrochemical Systems -- 4.1 Prelude: From Fundamentals to Biotechnology -- 4.2 Microbial Bioelectrochemical Systems (BESs) -- 4.2.1 The Archetype: Microbial Fuel Cells (MFCs) -- 4.2.2 Strength Through Diversity: Microbial Bioelectrochemical Systems -- 4.3 Bioelectrocatalysis: Microorganisms Catalyze Electrochemical Reactions -- 4.3.1 Energetic Considerations of Microbial Bioelectrocatalysis -- 4.3.1.1 Case Study: The Anodic Acetate Oxidation by Geobacteraceae -- 4.3.1.2 Case Study: The Cathodic Hydrogen Evolution Reaction (HER) -- 4.3.2 Microbial Electron Transfer Mechanisms -- 4.3.2.1 Direct Electron Transfer (DET) -- 4.3.2.2 Mediated Electron Transfer (MET) -- 4.3.2.3 Cathodic Electron Transfer Mechanisms -- 4.3.3 Microbial Interactions: Ecological Networks -- 4.3.3.1 Interspecies Electron Transfer and "Scavenging" of Redox-Shuttles -- 4.4 Characterizing Anodic Bio.lms by Electrochemical and Biological Means -- 4.4.1.1 Case Study: On the use of Cyclic Voltammetry -- 4.4.1.2 Case Study: Raman Microscopy -- References -- 5 Electrocapillarity of Solids and its Impact on Heterogeneous Catalysis -- 5.1 Introduction -- 5.2 Mechanics of Solid Electrodes -- 5.2.1 Outline - Surface Stress and Surface Tension -- 5.2.2 Solid Versus Fluid -- 5.2.3 Free Energy of Elastic Solid Surfaces -- 5.2.4 Deforming a Solid Surface -- 5.2.5 Case Study: Thought Experiment in Electrowetting -- 5.2.6 Capillary Equations for Fluids and Solids -- 5.2.7 Case Study: Molecular Dynamics Study of Surface-Induced Pressure -- 5.3 Electrocapillary Coupling at Equilibrium -- 5.3.1 Outline - Polarizable and Nonpolarizable Electrodes -- 5.3.2 Lippmann Equation and Electrocapillary Coupling Coefficient -- 5.3.3 Case Study: Cantilever-Bending Experiment in Electrolyte.
5.3.4 Important Maxwell Relations for Electrocapillarity -- 5.3.5 Electrocapillary Coupling During Electrosorption -- 5.3.6 Coupling Coefficient for Adsorption from Gas -- 5.3.7 Coupling Coefficient for the Langmuir Isotherm -- 5.3.8 Case Study: Strain-Dependent Hydrogen Underpotential Deposition -- 5.3.9 Coupling Coefficient for Potential of Zero Charge and Work Function -- 5.3.10 Empirical Data for the Electrocapillary Coupling Coefficient -- 5.4 Exploring the Dynamics -- 5.4.1 Outline -- 5.4.2 Cyclic Cantilever-Bending Experiments -- 5.4.3 Dynamic Electro-Chemo-Mechanical Analysis -- 5.5 Mechanically Modulated Catalysis -- 5.5.1 Outline -- 5.5.2 Phenomenology -- Distinguishing Capacitive from Faraday Current -- 5.5.3 Rate equations: Butler-Volmer kinetics -- 5.5.4 Rate Equations: Heyrowsky Reaction -- 5.6 Summary and Outlook -- References -- 6 Synthesis of Precious Metal Nanoparticles with High Surface Energy and High Electrocatalytic Activity -- 6.1 Introduction -- 6.2 Shape-Controlled Synthesis of Monometallic Nanocrystals with High Surface Energy -- 6.2.1 Electrochemical Route -- 6.2.1.1 Platinum -- 6.2.1.2 Palladium -- 6.2.2 Wet-Chemical Route -- 6.2.2.1 Platinum -- 6.2.2.2 Palladium -- 6.2.2.3 Gold -- 6.3 Shape-Controlled Synthesis of Bimetallic NCs with High Surface Energy -- 6.3.1 Surface Modification -- 6.3.1.1 Bi-Modified THH Pt NCs -- 6.3.1.2 Ru-Modified THH Pt NCs -- 6.3.1.3 Au-Modified Pt THH NCs -- 6.3.1.4 Pt-Modified Au Prisms with High-Index Facets -- 6.3.2 Alloy NCs -- 6.3.2.1 THH PdPt Alloy -- 6.3.2.2 HOH PdAu Alloy -- 6.3.3 Core-Shell Structured NCs -- 6.4 Concluding Remarks and Perspective -- References -- 7 X-Ray Studies of Strained Catalytic Dealloyed Pt Surfaces -- 7.1 Introduction -- 7.2 Dealloyed Bimetallic Surfaces -- 7.3 Dealloyed Strained Pt Core-Shell Model Surfaces.
7.4 X-Ray Studies of Dealloyed Strained PtCu3(111) Single Crystal Surfaces -- 7.5 X-Ray Studies of Dealloyed Strained Pt-Cu Polycrystalline Thin Film Surfaces -- 7.6 X-Ray Studies of Dealloyed Strained Alloy Nanoparticles -- 7.6.1 Bragg Brentano Powder X-Ray Diffraction (XRD) -- 7.6.2 In Situ High Temperature Powder X-Ray Diffraction (XRD) -- 7.6.3 Synchrotron X-Ray Photoemission Spectroscopy (XPS) -- 7.6.4 Anomalous Small Angle X-Ray Scattering (ASAXS) -- 7.6.5 Anomalous Powder X-Ray Diffraction (AXRD) -- 7.6.6 High Energy X-Ray Diffraction (HE-XRD) and Atomic Pair Distribution Function (PDF) Analysis -- 7.7 Conclusions -- References -- Index.
Abstract:
Catalysts speed up a chemical reaction or allow for reactions to take place that would not otherwise occur. The chemical nature of a catalyst and its structure are crucial for interactions with reaction intermediates. An electrocatalyst is used in an electrochemical reaction, for example in a fuel cell to produce electricity. In this case, reaction rates are also dependent on the electrode potential and the structure of the electrical double-layer. This work provides a valuable overview of this rapidly developing field by focusing on the aspects that drive the research of today and tomorrow. Key topics are discussed by leading experts, making this book a must-have for many scientists of the fi eld with backgrounds in different disciplines, including chemistry, physics, biochemistry, engineering as well as surface and materials science. This book is volume XIV in the series "Advances in Electrochemical Sciences and Engineering".
Local Note:
Electronic reproduction. Ann Arbor, Michigan : ProQuest Ebook Central, 2017. Available via World Wide Web. Access may be limited to ProQuest Ebook Central affiliated libraries.
Genre:
Electronic Access:
Click to View