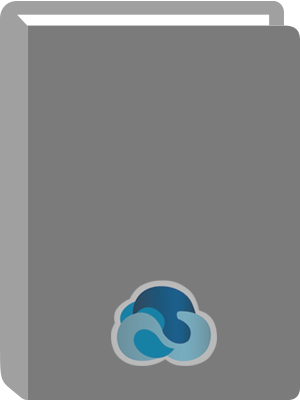
Optical Trapping and Manipulation of Neutral Particles Using Lasers : A Reprint Volume with Commentaries.
Title:
Optical Trapping and Manipulation of Neutral Particles Using Lasers : A Reprint Volume with Commentaries.
Author:
Ashkin, Arthur.
ISBN:
9789812774897
Personal Author:
Physical Description:
1 online resource (941 pages)
Contents:
Contents -- Preface -- I Introduction -- Chapter 1 Beginnings -- 1.1. Radiation Pressure Using Microwave Magnetrons -- 1.2. Runners and Bouncers -- 1.3. Back of the Envelope Calculation of Laser Radiation Pressure -- 1.4. First Observation of Laser Radiation Pressure -- 1.5. Observation of the First Three-Dimensional All-Optical Trap -- 1.6. Scattering Force on Atoms -- 1.7. Saturation of the Scattering Force on Atoms -- 1.8. Gradient (Dipole) Force on Atoms -- 1.9. Dispersive Properties of the Dipole Force on Atoms -- 1.10. Applications of the Scattering Force -- 1.11. "It's not Even Wrong!" -- 1.12. Optical Traps and the Prepared Mind -- II 1969-1979 -- Chapter 2 Optical Levitation -- 2.1. Levitation in Air -- 2.2. Scientific American Article of 1973 -- 2.3. Levitation with TEM01*Donut Mode Beams -- 2.4. Levitation of Liquid Drops -- 2.5. Radiometric or Thermal Forces -- 2.6. Levitation at Reduced Air Pressure -- 2.7. Feedback Damping of Levitated Particles and Automatic Force Measurement -- 2.8. Feedback Measurement of Axial Scattering Force -- 2.9. Feedback Force Measurement of High-Q Surface Wave Resonances -- 2.10. Measurement of Electric Forces by Feedback Control of Levitated Particles -- Chapter 3 Atom Trapping and Manipulation by Radiation Pressure Forces -- 3.1. Early Concepts and Experiments with Atoms -- 3.1.1. Deflection of atoms by the scattering force -- 3.1.2. Doppler cooling of atoms using the scattering force and fluctuational heating of atoms -- 3.1.3. Damping of macroscopic particles -- 3.1.4. Saturation of the gradient force on atoms -- 3.1.5. Optimum potential p for a given laser power -- 3.1.6. Conservative and nonconservative properties of the radiation pressure force components -- 3.1.7. Two-beam optical dipole traps for atoms.
3.1.8. Single-beam optical dipole trap or tweezer trap for atoms -- 3.1.9. Separate trapping and cooling beams and the Stark shift problem -- 3.1.10. First demonstration of the dipole force on atoms using detuned light -- 3.1.11. Origin of atom optics -- 3.2. Theoretical Aspects of Optical Forces on Atoms -- 3.2.1. Quantum theory of "The motion of atoms in a radiation trap" -- 3.2.2. Optical Stark shifts and dipole force traps for atoms -- 3.2.3. Optical dipole forces -- 3.2.4. Conservation of momentum in light scattering by atoms and sub-micrometer particles -- Chapter 4 Summary of the First Decades Work on Optical Trapping and Manipulation of Particies -- III 1980-1990 -- Chapter 5 Trapping of Atoms and Biological Particies in the 1980-1990 Decade -- 5.1. Optical Trapping and Cooling of Neutral Atoms in the Decade 1980-1990 -- 5.1.1. Slowing of atomic beams by the scattering force -- 5.1.2. Scattering force traps and the optical Earnshaw theorem -- 5.1.3. Arrival of Steve Chu at the Holmdel Laboratory -- 5.1.4. Planning for the first atom trapping experiment -- 5.1.5. Stable alternating beam scattering force atom traps -- 5.1.6. First demonstration of optical molasses and early work on an optical trap -- 5.1.7. Cooling below the Doppler limit of molasses and below the recoil limit -- 5.1.8. Evaporative cooling from optical dipole traps -- 5.1.9. First atom trapping experiment using the single-beam dipole trap -- 5.1.10. Proposal for stable spontaneous force light traps -- 5.1.11. Nature's comments on the first atom trapping experiment -- 5.1.12. The first experimental demonstration of a MOT -- 5.1.13. Radiation trapping in MOTs -- 5.1.14. Atom cooling below the Doppler limit -- 5.2. Trapping of Biological Particles -- 5.2.1. Artificial nonlinear media.
5.2.2. Trapping of submicrometer Rayleigh particles -- 5.2.3. Tweezer trapping of micrometer-sized dielectric spheres -- 5.2.4. Optical trapping and manipulation of viruses and bacteria -- 5.2.5. Optical alignment of tobacco mosaic viruses -- 5.2.6. Fixed particle arrays of tobacco mosaic viruses -- 5.2.7. Tweezer trapping of bacteria and "opticution" -- 5.2.8. Tweezer trapping of bacteria in a high-resolution microscope -- 5.2.9. Optical tweezers using infrared light from a Nd:YAG laser -- IV 1990-2006 -- IVA Biological Applications -- Chapter 6 General Biological Applications -- 6.1. Application of Tweezers to the Study of Bacteria -- 6.1.1. Bacteria flagella and bacterial motors -- 6.1.2. Optical manipulation of extremophilia -- 6.1.3. Pilus retraction powers bacterial twitching motility -- 6.1.4. Direct observation of extension and retraction of type-IV pili -- 6.1.5. A force-dependent switch reverses type-IV pilus retraction -- 6.1.6. Characterization of photodamage to Escherichia coli in optical traps -- 6.2. Use of UV Cutting Plus Tweezers to Study Cell Fusion and Chromosomes -- 6.3. Tweezer Manipulation of Live Sperm and Application to In Vitro Fertilization -- 6.4. Tweezer Study of the Immune Response of T-Lymphocytes -- 6.5. Adhesion of Influenza Virus to Red Blood Cells Using OPTCOL Technique -- 6.6. Mechanical Properties of Membranes Studied by Tether Formation Using Tweezers -- 6.7. Deformation of Single Cells by Light Forces -- 6.8. Artificial Gravity in Plants -- 6.9. Guiding of Neuronal Growth with Light -- 6.10. Self-Rotation of Red Blood Cells in Optical Tweezers -- Chapter 7 Use of Optical Tweezers to Study Singie Motor Molecules -- 7.1. In Vivo Force Measurement of Dynein in Giant Amoeba Reticulomyxa -- 7.2. Measurement of the Force Produced by Kinesin.
7.3. Resolution of the Stepping Motion of Kinesin on Microtubules by Interferometry -- 7.4. Observation of Single Stepwise Motion of Muscle Myosin-ll Molecules on Actin Using Feedback and Tweezers -- 7.5. Measurement of Diffusional Motion and Stepping in Actin-Myosin Interactions -- 7.6. Measurement of Myosin Step Size Using an Oriented Single-Headed Molecule -- 7.7. Forces on Smooth Muscle Myosin and Use of Fluorescently Labeled ATP with Total Internal Reflection Microscopy -- 7.8. Observation of Two-Step Behavior of Myosin I Using the Tweezer Dumbbell Technique -- 7.9. Study of Processive Class-V Myosins Using a Pair of Tweezer Traps -- 7.10. Force vs. Velocity Measurement on Kinesin Motor Molecules -- 7.11. Single Enzyme Kinetics of Kinesin -- 7.12. Kinesin Hydrolyses One ATP Molecule per 8 nm Step -- 7.13. Feedback Control of Tweezers: Force Clamps and Position Clamps -- 7.14. Study of Single Kinesin Molecules with a Force Clamp -- 7.15. Structural Measurements on Kinesin -- 7.16. Substeps within the 8 nm Step of the ATPase Cycle of Single Kinesin Molecules -- 7.17. Processivity of a Single-Headed Kinesin Construct C351 and the Brownian Ratchet -- 7.18. Myosin VI is a Processive Motor with a Large Step Size -- 7.19. Mapping the Actin Filament with Myosin -- 7.20. Development Regulation of Vesicle Transport in Drosophila Embryos: Forces and Kinetics -- 7.21. Dynein-Mediated Cargo Transport in Vivo: A Switch Controls Travel Distance -- 7.22. Kinesin Moves by an Asymmetric Hand-Over-Hand Mechanism -- Chapter 8 Applications to RNA and DNA -- 8.1. Observation of the Force of an RNA Polymerase Molecule as it Transcribes DNA -- 8.2. Force and Velocity Measured for Single Molecules of RNA Polymerase -- 8.3. Measurement of the Mechanical Properties of DNA Polymer Strands.
8.4. Measurement of Flexural Rigidity of Microtubule Fibers and Torsional Rigidity of Microtubules and Actin Filaments -- 8.5. Measurement of the Stretching of Double-and Single-Stranded DNA -- 8.6. Polymerization of RecA Protein on Individual dsDNA Molecules -- 8.7. Study of Elasticity of RecA-DNA Filaments with Constant Tension Feedback -- 8.8. Possible Role of Tweezers in DNA Sequencing -- 8.9. Study of the Structure of DNA and Chromatin Fibers by Stretching with Light Forces -- 8.10. Condensation and Decondensation of the Same DNA Molecule by Protamine and Arginine Molecules -- 8.11. Non-Mendelian Inheritance of Chloroplast DNA in Living Algal Cells Using Tweezers -- 8.12. Measurement of the Force and Mechanical Properties of DNA Polymerase with Optical Tweezers -- 8.13. Reversible Unfolding of Single RNA Molecules by Mechanical Force -- 8.14. Grafting of Single DNA Molecules to AFM Cantilevers Using Optical Tweezers -- 8.15. Structural Transition and Elasticity from Torque Measurements on DNA -- 8.16. Backtracking by Single RNA Polymerase Molecules Observed at Near-Base-Pair Resolution -- 8.17. Ubiquitous Transcriptional Pausing is Independent of RNA Polymerase Backtracking -- 8.18. RNA Polymerase can Track a DNA Groove During Promoter Search -- 8.19. The Bacterial Condensin MukBEF Compacts DNA into a Repetitive Stable Structure -- 8.20. Forward and Reverse Motion of RecBCD Molecules on DNA -- 8.21. Direct Observation of Base-Pair Stepping by RNA Polymersase -- Chapter 9 Study of the Mechanical Properties of Other Macromoiecules with Optical Tweezers -- 9.1. Stretching and Relaxation of the Giant Molecule Titin -- 9.2. Cell Motility of Adherent Cells Over an Extra-Cellular Matrix -- 9.3. Study of Forces that Regulate the Movement of Plasma Membrane Proteins.
9.4. Membrane Tube Formation from Giant Vesicles by Dynamic Association of Motor Proteins.
Abstract:
This important volume contains selected papers and extensive commentaries on laser trapping and manipulation of neutral particles using radiation pressure forces. Such techniques apply to a variety of small particles, such as atoms, molecules, macroscopic dielectric particles, living cells, and organelles within cells. These optical methods have had a revolutionary impact on the fields of atomic and molecular physics, biophysics, and many aspects of nanotechnology. In atomic physics, the trapping and cooling of atoms down to nanokelvins and even picokelvin temperatures are possible. These are the lowest temperatures in the universe. This made possible the first demonstration of Bose-Einstein condensation of atomic and molecular vapors. Some of the applications are high precision atomic clocks, gyroscopes, the measurement of gravity, cryptology, atomic computers, cavity quantum electrodynamics and coherent atom lasers. A major application in biophysics is the study of the mechanical properties of the many types of motor molecules, mechanoenzymes, and other macromolecules responsible for the motion of organelles within cells and the locomotion of entire cells. Unique in vitro and in vivo assays study the driving forces, stepping motion, kinetics, and efficiency of these motors as they move along the cell's cytoskeleton. Positional and temporal resolutions have been achieved, making possible the study of RNA and DNA polymerases, as they undergo their various copying, backtracking, and error correcting functions on a single base pair basis. Many applications in nanotechnology involve particle and cell sorting, particle rotation, microfabrication of simple machines, microfluidics, and other micrometer devices. The number of applications continues to grow at a rapid rate. The author is the discoverer of optical trapping and optical tweezers. With his
colleagues, he first demonstrated optical levitation, the trapping of atoms, and tweezer trapping and manipulation of living cells and biological particles. This is the only review volume covering the many fields of optical trapping and manipulation. The intention is to provide a selective guide to the literature and to teach how optical traps really work. Sample Chapter(s). Chapter 1: Beginnings (764 KB). Contents: Optical Levitation; Trapping of Atoms and Biological Particles in the 1980-1990 Decade; Use of Optical Tweezers to Study Single Motor Molecules; Origin of Tweezer Forces on Macroscopic Particles Using Highly Focused Beams; Rotation of Particles by Radiation Pressure; Microchemistry; Uses of Slow Atoms; Role of All-Optical Traps and MOTs in Atomic Physics; Feshbach Resonances; Vortices and Frictionless Flow in Bose-Einstein Condensates; Trapped Fermi Gases; and other papers. Readership: Researchers and students of atomic physics, molecular physics, biophysics and nanotechnology; historians of science.
Local Note:
Electronic reproduction. Ann Arbor, Michigan : ProQuest Ebook Central, 2017. Available via World Wide Web. Access may be limited to ProQuest Ebook Central affiliated libraries.
Genre:
Electronic Access:
Click to View