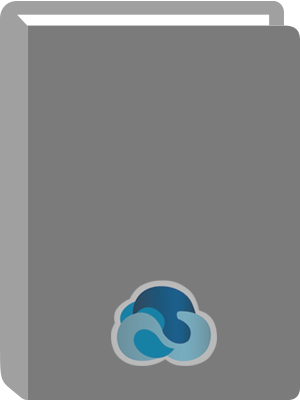
Handbook of Mechanical Nanostructuring, 2-Volume Set.
Title:
Handbook of Mechanical Nanostructuring, 2-Volume Set.
Author:
Aliofkhazraei, Mahmood.
ISBN:
9783527674978
Personal Author:
Edition:
1st ed.
Physical Description:
1 online resource (902 pages)
Contents:
Cover -- Contents -- List of Contributors -- Preface -- Volume 1 -- Part I Mechanical Properties of Nanostructured Materials -- Chapter 1 Mechanical Properties of Nanocrystalline Materials -- 1.1 Introduction -- 1.2 Static Properties -- 1.2.1 Tensile Behavior -- 1.2.2 Nanoindentation -- 1.3 Wear Properties -- 1.4 Fatigue Properties -- 1.5 Crack Behavior -- 1.6 Conclusions -- References -- Chapter 2 Superior Mechanical Properties of Nanostructured Light Metallic Materials and Their Innovation Potential -- 2.1 Introduction -- 2.2 Nanostructuring of Light Metallic Materials Using SPD Methods -- 2.3 Superior Mechanical Strength of NS Light Metals and Alloys -- 2.4 Fatigue Behavior of NS Light Metals -- 2.5 Innovation Potential and Application of the NS Light Metals and Alloys -- 2.6 Conclusions -- Acknowledgments -- References -- Chapter 3 Understanding the Mechanical Properties of Nanostructured Bainite -- 3.1 Introduction -- 3.2 NANOBAIN: Significant Extension of the Bainite Transformation Theory -- 3.2.1 Bainite Phase Transformation Thermodynamic Theory: Relevant Design Parameters -- 3.3 Microstructural Characterization of Nanostructured Bainitic Steels -- 3.4 Understanding the Advanced Bainitic Steel Mechanical Properties -- 3.4.1 Strength -- 3.4.2 Ductility -- 3.4.3 Toughness -- 3.5 Summary -- Acknowledgments -- References -- Chapter 4 Inherent Strength of Nano-Polycrystalline Materials -- 4.1 Introduction -- 4.2 High-field Tensile Testing -- 4.3 Tensile Strength of Nanosized Monocrystals -- 4.4 Inherent Strength of Bicrystals -- 4.5 Conclusions -- References -- Chapter 5 State-of-the-Art Optical Microscopy and AFM-Based Property Measurement of Nanostructure Materials -- 5.1 Introduction -- 5.1.1 Optical Microscopy -- 5.1.2 Near-Field Scanning Optical Microscopy -- 5.1.3 Atomic Force Microscopy.
5.2 Applications of Optical Microscopy and AFM -- 5.2.1 Applications of Optical Microscopy -- 5.2.2 Applications of Atomic Force Microscopy -- 5.3 New Developments of Optical Microscopy and AFM Techniques -- 5.3.1 Optical Microscopy-Based 3D Shape Reconstruction -- 5.3.1.1 Defocus Imaging Model -- 5.3.1.2 New Shape Reconstruction Method -- 5.3.1.3 Experimental Results -- 5.3.2 AFM Based Elasticity Imaging and Height Compensation Method -- 5.3.2.1 Compression Effect -- 5.3.2.2 Surface Characteristics Measurement -- 5.3.2.3 Experiments with MWCNTs and Graphenes -- 5.4 Conclusion -- References -- Chapter 6 Strength and Electrical Conductivity of Bulk Nanostructured Cu and Cu-Based Alloys Produced by SPD -- 6.1 Introduction -- 6.2 Microstructure and Strength and Electrical Conductivity of Bulk Nanostructured Cu Produced by SPD -- 6.2.1 Microstructure -- 6.2.2 Strength -- 6.2.2.1 Electrical Conductivity -- 6.3 Bulk Nanostructured Precipitation-Hardenable Cu-Cr Alloys from SPD -- 6.3.1 Bulk Nanostructured Precipitation-Hardenable Cu-Cr Alloys Produced by High-Pressure Torsion (HPT) -- 6.3.1.1 Microstructure -- 6.3.1.2 Strength and Electrical Conductivity -- 6.3.2 Bulk Nanostructured Precipitation-Hardenable Cu-Cr Alloys Produced by Equal-Channel Angular Pressing (ECAP) -- 6.3.2.1 Microstructure -- 6.3.2.2 Strength -- 6.3.2.3 Electrical Conductivity -- 6.4 Bulk Nanostructured Cu-Cr In Situ Fibrous Composites Produced by SPD -- 6.4.1 Microstructure -- 6.4.2 Strength -- 6.4.3 Electrical Conductivity -- 6.5 Perspectives for Industrial Applications of SPD to Produce Bulk Nanostructured Cu and Cu-Based Alloys with High Strength and High Electrical Conductivity -- 6.6 Conclusion -- Acknowledgements -- References.
Chapter 7 Mechanical Properties and Dislocation Boundary Mechanisms during Equal-Channel Angular Pressing (ECAP) -- 7.1 Introduction -- 7.2 Strength Contributions to Yield Stress -- 7.2.1 Aluminum Matrix Term -- 7.2.2 Solid Solution Strengthening Contribution Coming from the Dissolved Alloying Elements -- 7.2.3 Secondary-Phase Particles, Dispersoids, and Intermetallic Phase Strengthening -- 7.2.4 Dislocation Strengthening Generated by ECAP -- 7.2.5 Strengthening Contribution Due to Texture Evolution with ECAP Straining -- 7.3 Model Validation: Case Study -- 7.3.1 Solid Solution Strengthening -- 7.3.2 Secondary-Phase, Dispersoid, and Intermetallic-Phase Particle Strengthening -- 7.3.3 Dislocation Boundary Strengthening -- 7.3.4 Strengthening Due to Texture Evolution Induced by ECAP -- 7.3.5 Grain Boundary Sliding and Twinning -- 7.3.6 Models of Strengthening Contribution Combination -- 7.4 General Remarks and Prospects -- References -- Chapter 8 Mechanical Properties of Nanoparticles: Characterization by In situ Nanoindentation Inside a Transmission Electron Microscope -- 8.1 Introduction -- 8.2 In situ TEM Nanoindentation Developments -- 8.2.1 Description of the Sample Holder -- 8.2.2 Sample Geometry -- 8.3 Examples of In situ Nanoindentation Tests on Nanoparticles -- 8.3.1 Clusters -- 8.3.2 Isolated Nanoparticles -- 8.4 Data Processing -- 8.4.1 Contrast Imaging -- 8.4.2 Load-Displacement Curve Processing -- 8.4.3 Effect of the Electron Beam -- 8.5 Interest of Simulation on the Data Processing -- 8.6 Conclusion -- References -- Chapter 9 Improved Mechanical Properties by Nanostructuring - Specific Considerations under Dynamic Load Conditions -- 9.1 Introduction -- 9.2 General Considerations for Nanostructured Bulk Materials -- 9.2.1 The Hall-Petch Relation and Archard's Law -- 9.2.2 Activation Volume.
9.2.3 Quasistatic and Dynamic Testing of n-Nickel -- 9.3 Nanoparticle-Strengthened Nanometal-Matrix Composites -- 9.3.1 Hardening Mechanisms for Particle-Strengthened MMCs -- 9.3.2 Testing of n-Ni/n-Al2O3 MMCs -- 9.3.2.1 Wear Behavior of MMCs -- 9.3.2.2 Vickers Hardness of MMCs -- 9.3.2.3 Quasistatic and Dynamic Compression Tests on n-Nickel and MMCs -- 9.3.2.4 Conclusions on Properties of n-Nickel versus MMCs -- 9.4 Improved Mechanical Properties by Nanostructured Coatings -- 9.4.1 Mechanical Properties of n-Ni/Al Hybrid Metal Foams -- 9.5 Conclusion -- References -- Chapter 10 Mechanical Properties of Bio-Nanostructured Materials -- 10.1 Introduction -- 10.2 Types of Nanostructured Composites -- 10.3 Surface Effects -- 10.4 Biopolymer Nanocrystals and the Benefits of Hydrogen Bonding -- 10.4.1 Keratins -- 10.4.2 Chitin -- 10.4.3 Collagen -- 10.4.4 Spider Silk -- 10.5 Nanointerlocking Mechanisms -- 10.5.1 Surface Protuberances -- 10.5.2 Nanobridges -- 10.5.3 Interlocking nanofibres -- 10.5.4 Nanosutures and Fractal Surfaces -- 10.6 Methods for Determining the Nanomechanical Properties of Materials -- 10.6.1 Atomic Force Microscopy -- 10.6.2 Nanoindentation -- 10.6.3 Atomistic Modeling -- 10.6.3.1 Modeling of Atom-Atom Interactions -- 10.6.3.2 Molecular Dynamics -- 10.6.3.3 Lattice Energy (LE) Calculations -- 10.6.3.4 Monte Carlo Simulations -- 10.7 Conclusions -- References -- Part II Mechanical Nanostructuring Methods -- Chapter 11 SPD Processes - Methods for Mechanical Nanostructuring -- 11.1 Introduction -- 11.2 Classification of SPD Methods -- 11.2.1 Discontinuous SPD Processes -- 11.3 HPT (High-Pressure Torsion) -- 11.4 ECAP (Equal-Channel Angular Pressing) -- 11.5 Development of the ECAP Technique -- 11.5.1 Continuous SPD Processes -- 11.6 HPT Development -- 11.7 ECAP Development.
11.8 FEM Simulation of SPD Processes -- 11.9 Materials for SPD Techniques -- 11.9.1 Metals with Low Melting Points -- 11.9.2 Metals with Medium Melting Points -- 11.9.3 Metals with High Melting Points -- 11.10 Conclusion -- Acknowledgments -- References -- Chapter 12 Mechanical Alloying/Milling -- 12.1 Introduction -- 12.2 History and Development -- 12.3 Milling Process -- 12.4 Mechanism of Mechanical Alloying/Milling -- 12.5 Process Variables -- 12.5.1 Type of Mill -- 12.5.1.1 Attritor -- 12.5.1.2 SPEX Shaker Mill -- 12.5.1.3 Planetary Mill -- 12.5.2 Milling Medium -- 12.5.3 Milling Speed -- 12.5.4 Milling Time -- 12.5.5 Ball-to-Powder Weight Ratio (BPR) -- 12.5.6 Extent of Filling the Vial -- 12.5.7 Milling Atmosphere -- 12.5.8 Process Control Agents -- 12.5.9 Temperature for Milling -- 12.5.10 Contamination during Milling -- 12.6 Summary -- References -- Chapter 13 Equal-Channel Angular Pressing (ECAP) -- 13.1 Introduction -- 13.2 Die Design and Modifications -- 13.3 Influence of External and Internal Parameters -- 13.3.1 Influence of External Parameters -- 13.3.1.1 Channel Angle and Corner Angle -- 13.3.1.2 Friction -- 13.3.2 Influence of Internal Parameters -- 13.3.2.1 Influence of Strain Hardening -- 13.3.2.2 Temperature -- 13.3.2.3 Strain Rate Sensitivity -- 13.4 ECAP of Aluminum and Its Alloys -- 13.4.1 Mechanical Properties -- 13.4.2 Corrosion Properties -- 13.5 ECAP of Copper -- 13.5.1 Mechanical Properties -- 13.5.2 Corrosion Properties -- 13.5.3 Electrical Conductivity -- 13.5.4 Fatigue Properties -- 13.6 ECAP of Titanium -- 13.6.1 Mechanical Properties -- 13.6.2 Wear Properties -- 13.6.3 Corrosion Properties -- 13.6.4 Biocompatibility -- 13.7 ECAP of Magnesium and Steels -- 13.8 ECAP for Consolidation of Powders -- 13.9 Suitability for Large-Scale Production -- 13.10 Summary -- References.
Chapter 14 Severe Shot Peening to Obtain Nanostructured Surfaces: Process and Properties of the Treated Surfaces.
Abstract:
The nanostructuring of materials is a versatile route particularly well-suited to the fabrication of metallic materials for engineering applications with desired properties, for example, increased corrosion and temperature resistance, enhanced performance under mechanical loads or the long-term shape preservation of workpieces. This ready reference provides in-depth information on both the bottom-up and the top-down approaches to the synthesis and processing of nanostructured materials. The focus is on advanced methods of mechanical nanostructuring, such as severe plastic deformation, including high pressure torsion, equal channel angular processing, cyclic extrusion compression, accumulative roll bonding, and surface mechanical attrition treatment. As such, the contents are inherently application-oriented, with the methods presented able to be easily integrated into existing production processes. In addition, the structure-property relationships and ways of influencing the nanostructure are reviewed in detail. The whole is rounded off by a look at future directions, followed by an overview of applications in various fields of structural and mechanical engineering. With its solutions for the successful processing of complex shapes and large-scale specimens, this is an indispensable tool for purposeful materials design.
Local Note:
Electronic reproduction. Ann Arbor, Michigan : ProQuest Ebook Central, 2017. Available via World Wide Web. Access may be limited to ProQuest Ebook Central affiliated libraries.
Subject Term:
Genre:
Electronic Access:
Click to View