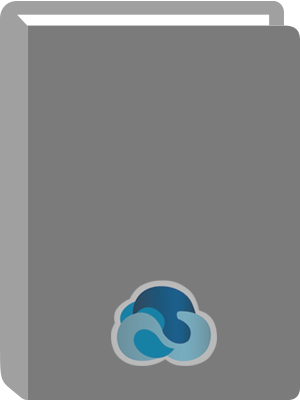
Strong Light-Matter Coupling : From Atoms to Solid-State Systems.
Title:
Strong Light-Matter Coupling : From Atoms to Solid-State Systems.
Author:
Auffeves, Alexia.
ISBN:
9789814460354
Personal Author:
Physical Description:
1 online resource (303 pages)
Contents:
Contents -- Preface -- Chapter 1. Cavity QED in Atomic Physics -- 1. Foreword -- 2. Introduction -- 3. A spin and a spring -- 3.1. Experimental set-up -- 3.2. Atom-field interaction -- 3.3. Field relaxation -- 4. Ideal QND measurement of the photon number: The quantum jumps of light -- 5. Monitoring the decoherence of mesoscopic quantum superpositions -- 6. An experiment on quantum feedback -- 7. Reservoir engineering -- 8. Conclusion and perspectives -- References -- Chapter 2. Exciton-Polaritons in Bulk Semiconductors -- 1. Introduction -- 2. Excitons and polaritons in bulk semiconductors -- 2.1. Wannier-Mott excitons, oscillator strength, exchange interaction -- 2.2. Exciton-polaritons: semiclassical theory -- 2.3. Exciton-polaritons: quantum theory -- 3. Excitons and polaritons in two dimensions -- 3.1. Exciton and polariton effects in quantum wells -- 3.2. Radiative recombination of excitons in 2D, 1D, 0D -- 3.3. Photon confinement in planar microcavities -- 3.4. Polaritons in planar microcavities -- 3.5. Polaritons in waveguide-embedded photonic crystals -- 4. From three to zero dimensions -- 4.1. Polaritons in pillar microcavities -- 4.2. Quantum dots in three-dimensional microcavities: Strong coupling of 0D excitons with 0D photons -- 4.3. Exciton-photon coupling in different dimensionalities -- 5. Conclusions -- References -- Chapter 3. Experimental Circuit QED -- Introduction -- 1. Basics of circuit QED -- 1.1. Basics of Josephson junctions and SQUIDs -- 1.2. Josephson resonators in the quantum regime -- 1.2.1. Linear resonators -- 1.2.2. Nonlinear resonators -- 1.2.3. Transmon qubit -- 1.2.4. Flux-tunable resonators -- 1.3. Transmon coupled to a resonator: Circuit QED -- 1.3.1. Coupling hamiltonian -- 1.3.2. Dispersive regime -- 2. Experimental methods in circuit QED -- 2.1. Temperatures -- 2.2. Measuring a linear resonator.
2.3. Measuring a Josephson resonator -- 2.4. Qubit readout in circuit QED -- 3. Conclusion -- References -- Chapter 4. Quantum Open Systems -- 1. Introduction -- 2. Damped cavity/resonator -- 2.1. Basic elements -- 2.1.1. The cavity/resonator mode and damping rate -- 2.1.2. The multi-mode reservoir (external electromagnetic field) -- 2.1.3. Free field and photon flux units -- 2.1.4. Interaction and coupling strength -- 2.2. The Lindblad master equation -- 2.3. The quantum Langevin equation -- 2.4. Input and output fields -- 2.5. Other examples -- 2.5.1. Damped cavity/resonator with a thermal (chaotic) input -- 2.5.2. Two-state atom -- 2.6. What's in a master equation? -- 2.6.1. Diagonal matrix elements -- 2.6.2. Initial coherent state -- 2.6.3. Coherently driven cavity -- 3. Correlation functions and quantum-classical correspondence -- 3.1. Quantum regression -- 3.1.1. By solving the master equation twice -- 3.1.2. In the manner of Lax -- 3.2. Example: Hanbury Brown and Twiss -- 3.3. The Glauber-Sudarshan P representation -- 3.3.1. The Fokker-Planck equation -- 3.3.2. The Langevin equation -- 3.4. Quantum-classical correspondence -- 3.4.1. Backaction: conditional states and 'a priori' paths -- 3.4.2. Elements of photoelectron counting theory -- 3.4.3. Classical versus nonclassical light -- 4. Quantum trajectories: Don't trace, disentangle -- 4.1. Sketch #1: Labeling kets with "records" -- 4.2. Sketch #2: Familiar and unfamiliar expansions of ρ -- 4.2.1. Bohr-Einstein quantum jumps: Unconditional expansion of ρ -- 4.2.2. Record probabilities and expansion in conditional states -- 4.2.3. From the quantum master equation -- 4.2.4. Extension to pure states -- 5. Quantum trajectories and photoelectron counting -- 5.1. Photoelectron counting records and conditional states -- 5.1.1. Master equation unraveling -- 5.1.2. Record probability density.
5.2. Sum over records in S and in S R -- 5.3. Monte Carlo algorithm for jump trajectories -- 5.3.1. Following paths: Bayesian inference -- 5.3.2. A simple Monte Carlo procedure -- 5.4. Examples -- 5.4.1. Resonance fluorescence -- 5.4.2. Electron shelving -- 5.4.3. Coherent states and superpositions of coherent states -- 6. Cascaded systems -- 6.1. Model -- 6.1.1. Hamiltonian -- 6.1.2. Inputs and outputs -- 6.2. The Lindblad master equation -- 6.3. Quantum trajectory unraveling -- 6.3.1. Jump operator and non-Hermitian Hamiltonian -- 6.3.2. Example: Initial coherent state -- Acknowledgments -- References -- Chapter 5. Basic Concepts in Quantum Information -- 1. Introduction and overview -- 1.1. Classical information and computation -- 1.2. Quantum information -- 1.3. Quantum algorithms -- 2. Introduction to quantum information -- 3. Quantum money and quantum encryption -- 3.1. Classical and quantum encryption -- 4. Entanglement, bell states and superdense coding and grover search and teleportation -- 4.1. Quantum dense coding -- 4.2. No-cloning theorem revisited -- 4.3. Quantum teleportation -- 5. Quantum error correction -- 6. Introduction to circuit QED -- 6.1. Electromagnetic oscillators -- 6.2. Superconducting qubits -- 6.3. The cooper pair box and the transmon qubit -- 6.4. Circuit QED: qubits coupled to resonators -- 7. Summary -- Acknowledgments -- References -- Chapter 6. Cavity Polaritons: Crossroad Between Non-Linear Optics and Atomic Condensates -- 1. Introduction -- 2. Cavity polaritons: exciton-photon strong coupling regime -- 3. Laterally con.ning cavity polaritons -- 3.1. One dimensional microcavities -- 3.2. Zero dimensional cavities: single and coupled micropillars -- 4. Polariton condensation -- 4.1. Resonantly driven polariton condensates -- 4.2. Polariton condensation under non resonant excitation.
4.3. Polariton interaction with the excitonic reservoir -- 4.4. Polariton condensation in a periodic potential: interplay between reservoir interaction and self-interaction -- 5. Josephson effects in coupled micropillars -- 6. Ring structures: engineering geometric phases -- 6.1. The polariton benzene molecule -- 6.2. Spin-orbit coupling -- 7. Polariton lattices -- 8. Conclusion -- Acknowledgments -- References -- Chapter 7. Quantum Plasmonics -- 1. Introduction -- 2. Introduction to surface plasmons -- 3. Efficient coupling between SPs and quantum emitters -- 4. Single-photon nonlinear optics -- 5. State-of-the-art and outlook -- References -- Chapter 8. Quantum Polaritonics -- 1. Electronic excitation in semiconductor -- 2. Linear and nonlinear dynamics -- 3. Entangled photon pairs from the optical decay of biexcitons -- 4. The picture of interacting polaritons -- 5. Noise and environment: Quantum Langevin approach -- 6. Quantum complementarity of cavity polaritons -- 7. Emergence of entanglement out of a noisy environment: The case of microcavity polaritons -- 7.1. Coherent and incoherent polariton dynamics -- 7.2. Results -- 8. Quantum plasmonics: Individual quantum emitters coupled to metallic nanoparticles -- 8.1. QE-MNP hybrid artificial molecule -- 8.2. Plexcitons (nanopolaritons) with a single quantum emitter -- 9. Outlook -- References -- Chapter 9. Optical Signal Processing with Enhanced Nonlinearity in Photonic Crystals -- 1. Introduction: All optical processing -- 2. The optical transistor -- 3. The optical memory -- 4. Nonlinear waves on chip -- 5. Conclusions -- Acknowledgements -- References.
Abstract:
The physics of strong light-matter coupling has been addressed in different scientific communities over the last three decades. Since the early eighties, atoms coupled to optical and microwave cavities have led to pioneering demonstrations of cavity quantum electrodynamics, Gedanken experiments, and building blocks for quantum information processing, for which the Nobel Prize in Physics was awarded in 2012. In the framework of semiconducting devices, strong coupling has allowed investigations into the physics of Bose gases in solid-state environments, and the latter holds promise for exploiting light-matter interaction at the single-photon level in scalable architectures. More recently, impressive developments in the so-called superconducting circuit QED have opened another fundamental playground to revisit cavity quantum electrodynamics for practical and fundamental purposes. This book aims at developing the necessary interface between these communities, by providing future researchers with a robust conceptual, theoretical and experimental basis on strong light-matter coupling, both in the classical and in the quantum regimes. In addition, the emphasis is on new forefront research topics currently developed around the physics of strong light-matter interaction in the atomic and solid-state scenarios.
Local Note:
Electronic reproduction. Ann Arbor, Michigan : ProQuest Ebook Central, 2017. Available via World Wide Web. Access may be limited to ProQuest Ebook Central affiliated libraries.
Genre:
Electronic Access:
Click to View